Author | Affiliation |
---|---|
Donald M. Dawes, MD | Lompoc Valley Medical Center, Lompoc, CA |
Jeffrey D. Ho, MD | Hennepin County Medical Center, Minneapolis, MN |
Robert F. Reardon, MD | Hennepin County Medical Center, Minneapolis, MN |
James D. Sweeney, PhD | Florida Gulf Coast University, Fort Myers, FL |
James R. Miner, MD | Hennepin County Medical Center, Minneapolis, MN |
ABSTRACT
Introduction:
Law enforcement and military personnel use electronic control devices to control non-compliant and actively resistive subjects. The TASER® Shockwave is a new electronic control device designed specifically as an area denial device capable of delivering multiple simultaneous discharges. This is the first study to examine the effects of multiple simultaneous device discharges in humans.
Methods:
Volunteers were exposed to multiple (two to three), simultaneous 5-second discharges from the Shockwave device to the chest, back, chest to abdomen, or thighs. Blood was analyzed before and after discharge for pH, lactate, potassium, creatine kinase (CK), and troponin. Continuous spirometry was performed before, during, and after the discharge. In addition, electrocardiograms (ECGs) before and after discharge were recorded, and echocardiography was used to determine the rhythm during discharge.
Results:
Small elevations of lactate occurred. Moderate increases in CK at 24 hours occurred and appeared to be related to the number of simultaneous discharges. There was a trend to a decrease in minute ventilation in the volunteers exposed to two simultaneous discharges, but it did not reach statistical significance. ECG changes only reflected an increase in vagal tone, and there was no evidence of capture by echocardiography. Five-second, simultaneous, multiple exposures to the TASER Shockwave device were reasonably tolerated by our human volunteers.
Conclusion:
Our study suggests that this device may have a reasonable risk/benefit ratio when used to protect an area from a threat.
INTRODUCTION
Law enforcement and military personnel use electronic control devices to control non-compliant and actively resistive subjects. The TASER® X26 is the most commonly used handheld electronic control device. It fires two nitrogen-propelled probes up to 25 feet to cause incapacitation of the target.
The TASER® Shockwave is a new electronic control device designed specifically as an area denial device. It operates as a remotely triggered stationary platform that deploys multiple standard TASER cartridges (the same cartridges used in the TASER X26) to saturate an area and immobilize multiple subjects or a moving single subject. As with other electronic control devices, the probes deliver a charge that stimulates the motor neurons, which causes involuntary sub-tetanic muscle contraction and subject incapacitation. The charge is delivered for five seconds, and can be repeated multiple times. This device is available to law enforcement and military authorities to prevent unwanted persons from entering protected areas. The human physiologic effects of the TASER X26 have been previously studied, but this is the first study to examine the effects of multiple, simultaneous device discharges.
METHODS
This was a prospective, observational study of human subjects. The subjects were a convenience sample of law enforcement officers receiving an exposure as part of a TASER training course. The local institutional review committee approved the study. Subjects provided informed consent and completed a medical screening questionnaire that was reviewed by a study physician. The questionnaire was used to collect demographic data such as age, height and weight, past medical history, and current medications. There were no specific exclusion criteria, but all subjects had to be at full duty status with their agencies. In addition, the ultimate safety of the subjects was the responsibility of the supervising physician on that study day and that physician could exclude subjects based on a review of their medical questionnaire. Advanced age or orthopedic problems that could make the subject unnecessarily at risk from the muscle contractions could have been reasons for exclusion, as well as recent cardiovascular events. Any subjects excluded and the reason for exclusion would be declared. Subjects were given a TASER X26, donated by TASER International, as compensation for their participation.
Human volunteers were exposed to multiple (two to three), simultaneous 5-second discharges from the Shockwave device as determined by the training instructors. The conductive wires were taped into conducting gel on the skin surface. To simulate field conditions where subjects are 5–10 feet from the device placed on level ground, the training instructors decided to do four types of exposures with a 6–10 inch horizontal spread between pairs of electrodes and a 9–18 inch vertical spread between the top and bottom electrodes in a pair:
-
Chest to abdominal area – Three simultaneous devices and a smaller spread between pairs of electrodes (simulating close distance to the device when it is activated)
-
Thigh – Two simultaneous devices and a smaller spread between pairs of electrodes (simulating close distance and the likelihood that the probes fired from the middle cartridge would miss due to separation between the legs)
-
Chest – Two simultaneous devices and a larger spread between pairs of electrodes (to simulate the expected dispersion at a farther distance)
-
Back – Two simultaneous devices and a larger spread between pairs of electrodes (to simulate the expected dispersion at a farther distance)
Blood was drawn before, immediately after (within 1–2 minutes), and at 24 hours post exposure, and was analyzed for pH, lactate, potassium, and troponin on the Abbott Point-of-Care i-STAT® (East Windsor, NJ). Creatine kinase (CK) values were also determined by LabCorp independent testing (Scottsdale, AZ). A breath-by-breath analyzer was used to collect continuous respiratory data (Med Graphics CPX Ultima®, Minneapolis, MN). Electrocardiograms (ECGs) were measured before and immediately (within one minute) after the exposures (Welch Allyn Cardio-perfect System®, Skaneateles Falls, NY). A blinded cardiologist read the ECGs.
In a convenience sample (based on the availability of the echocardiographer), a non-blinded emergency physician expert in ultrasonography performed limited echocardiography using a Sonosite (Bothell, WA) M-Turbo portable ultrasound device with a P21x 5-1 MHz 21-mm broadbandphased array probe. Heart rate and rhythm were obtained before, during, and after the exposure. The echocardiographer kept the probe in place continuously so the heart could be observed immediately before and immediately after the exposure, although pre-exposure heart rates were recorded in the minute before the exposure, the heart rates during the exposure were recorded at the end of the 5-second exposure, and the post-exposure heart rates were recorded in the minute after exposure. E and A waves viewed in the continuous M-mode, using the parasternal long axis view through the anterior leaflet of the mitral valve, were used as evidence for sinus rhythm. The E wave corresponds to the mitral valve opening with passive filling of the left ventricle. The A wave corresponds to the mitral valve opening with atrial contraction.
Subjects had percentage of body fat measured by a commercial skin resistance analyzer (Omron Fat Loss Monitor HBF-306, Omron Healthcare, Inc., Bannockburn, Illinois). Subjects also had blood pressure and heart rate measured before and immediately (within 1–2 minutes) after the exposure (Nonin 2120, Nonin Medical, Inc., Plymouth, MN). Subjects were told not to engage in physical exertion for 48 hours before exposure and until after the final blood draw at 24 hours post exposure.
Data were entered into an Excel spreadsheet (Microsoft Corp, Redmond, WA) and exported into STATA 10.0 (Stata Corp, College Station, TX). Descriptive statistics were used where appropriate. Laboratory values were compared to baseline using Wilcoxon Sign Rank tests. Values between the 2- and 3-exposure groups were compared using Wilcoxon rank sum tests.
RESULTS
Sixteen subjects were enrolled: 13 males and 3 females. No subject was excluded based on review of his or her medical screening questionnaire.
Eight subjects received two simultaneous exposures; three of these had chest exposures with 15–18 inch vertical spreads and 10-inch horizontal spreads of electrodes, and three had back exposures with 14–18 inch vertical spreads and 9–10 inch horizontal spreads of electrodes. The remaining two had thigh exposures with 9-inch vertical spreads on each thigh.
Eight subjects received three simultaneous exposures on the lower chest (five inches below the nipple line) to lower abdomen with 9-inch vertical spreads and 6-inch horizontal spreads between electrodes.
The mean age of all subjects was 33 (range 19–57). The mean body mass index (based on stated heights and weights) was 27.9 (range 18.8–40.5). The mean percentage body fat was 23.9 (range 13–39.6). Demographics of the subjects are presented in Table 1. Other than some minor muscle soreness, there were no adverse outcomes reported. Several subjects even engaged in exercise routines that same day or the next day (compliance was variable for the physical exertion requirement: subjects 8, 9, 11, and 16 exercised moderately both the day of the exposure and the day after, prior to the repeat testing).
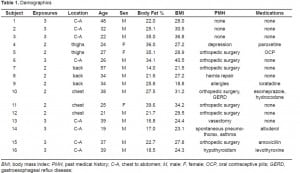
The blood results are presented in Table 2. No statistically or clinically important changes in pH occurred. Small elevations of lactate occurred. Moderate increases in CK occurred at 24 hours and this seemed to be related to the number of simultaneous exposures. No clinically important or statistically significant changes in potassium occurred. Troponin remained within the reference range for the i-STAT device for the 24 hours.
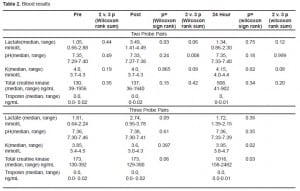
The breathing results are presented in Table 3. There was a trend to a decrease in minute ventilation in the subjects with chest or back exposures (two simultaneous exposures), although it did not reach statistical significance.
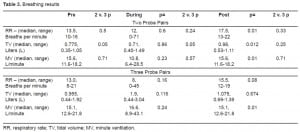
The ECG results are presented in Table 4. Generally, the changes only reflected some increased vagal tone after the discharge. There were no important rhythm changes. One subject had non-specific inferior T wave changes. His “before” and “after” ECGs are in Figure 1.
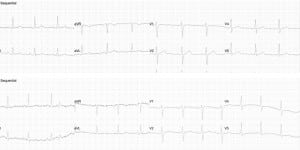
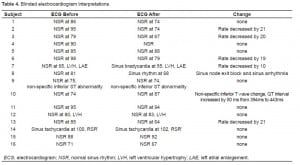
The echocardiography results are presented in Table 5. Four of the subjects had chest-to-abdomen exposures, and two had thigh exposures. In four of the six subjects, the echocardiographer was able to determine that the rhythm during discharge was sinus. In two subjects, one with thigh exposures and one with chest-to-abdomen exposures, motion artifact precluded this. One of these subjects (the thigh exposures) had a heart rate during the exposure of 79, not suggesting electrical capture. The other subject had a heart rate that was not a divisor of the 19 pulses per second, making capture less likely (4:1 capture for one device would give a rate of 143). The exposure locations are shown schematically in Figure 2. Vital signs are presented in Table 6.
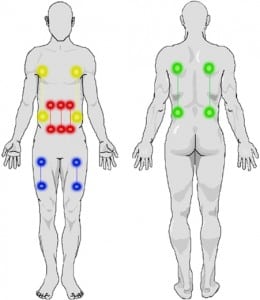
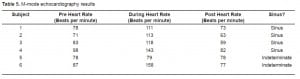
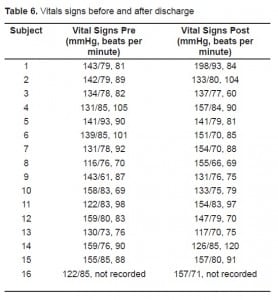
DISCUSSION
The TASER Shockwave, like the TASER X26, fires two probes from a single cartridge. Each probe pair forms an electric circuit (if one probe misses the intended target, the device cannot discharge through that cartridge). Depending on the configuration, the Shockwave can fire a large number of cartridges at one time, saturating an area and incapacitating a single moving subject or multiple subjects. Because of the trajectory of the fired probe pairs, it is unlikely that more than three electrical circuits would be completed on a single target. Because the electrical circuit is the same as the TASER X26, and this has been previously studied with single deployments, we elected to only study the effects of 2–3 simultaneous discharges. Spreads were decided by the trainers and were based on trajectory determined by TASER International for a ground-level deployed device.
There has been controversy in the lay press and medical literature about the use of conducted electrical weapons and temporally associated in-custody deaths. Amnesty International claims that these devices have caused more than 300 deaths.1 Several animal studies have shown that the TASER X26 can electrically capture the myocardium when discharged on the thorax. In Nanthakumar et al.,2 capture occurred in 78% of thoracic discharges. There was one episode of ventricular fibrillation in this study, but only after infusion of epinephrine. In Walter et al.,3 capture occurred in 100% of thoracic discharges. There was one episode of ventricular fibrillation in this study.
These results have not been reproduced in human studies. In Ho et al.,4 37 subjects received a 15-second TASER X26 exposure to the chest after a maximal exercise regimen. Mean heart rates were 86, 153, 140, and 115 pre-exercise, post-exercise, during-TASER X26 exposure, and post-TASER exposure, respectively, as determined by echocardiography. In half of the subjects, sinus rhythm was apparent by echocardiography. In a second study,5 34 subjects received a 10-second exposure in the cardiac axis. Mean heart rates were 106, 123, and 94 pre-TASER X26 exposure, during TASER exposure, and post-TASER exposure, respectively. In more than half of the subjects, sinus rhythm was apparent by echocardiography. Neither of these studies determined there was any evidence of myocardial capture by echocardiography. In a study by Jauchem,6 the swine had respiratory arrest during each discharge. However, that data has also been contradicted by human studies.7
It would be expected that skeletal muscle activation would vary as a function of electrode locations and spread, as well as numbers of electrodes activated (i.e., two versus three cartridges). Any muscle groups activated by both devices in the two exposure group (i.e., muscles within the effective “capture zone” of both device electrodes) will effectively receive a train of stimuli at 38 pulses per second (twice the normal 19 pulses per second of the TASER X26); muscles captured by all electrode pairs for the three-exposure group would receive 57 pulses per second of stimulation (three times 19 pulses per second). Maximal physiological firing rates of human motor neurons vary considerably dependent upon size-related properties of the neurons and the skeletal muscle fibers they innervate, but in general such maximal discharge rates are below 40 pulses per second for mixed fiber-type muscle groups, such as those of the torso and extremities.8 Forces and torques elicited by electrical stimulation of human skeletal muscles rise considerably as a function of frequency, becoming well tetanized by 38 pulses per second (our two-exposure group) with some expected additional fusion and peak force generation at 57 pulses per second (our three-exposure group).9
In our study, we found that there were small elevations of lactate immediately post-exposure. The subjects who had two devices, and therefore the larger spreads, had significantly larger increases in lactate compared to the subjects who had three devices (p<0.06). This suggests that the dominant factor in correlating to overall level of muscle activation with this marker may be related to electrode location and spread rather than number of devices. There was no significant, either statistical or clinical, change in pH. Our results were similar to other authors studying single-device exposures.10 In a study by Gass et al.,11 venous lactate increased to 14.2 mmol/L after a maximal exercise regimen on a treadmill in trained subjects. The changes in lactate in our study are small by comparison. We observed only moderate increases in CK at 24 hours for the majority of subjects across both exposure groups. A previous study by Ho et al.12 examined the CK changes after a 5-second TASER X26 discharge to the back (large spread). This study found that the mean CK value was elevated to a level of 242.3 U/L at 24 hours. In our current study, the median CK at 24 hours was somewhat higher in the three-exposure group compared to the two-exposure group (p<0.2). This may be explained by the fact that CK is a marker of rhabdomyolysis (muscle damage), not necessarily muscle mass activated, and in the three-exposure group, the pulses per second from the three devices (19 x 3) could be slightly more damaging than from the two devices (19 x 2). In a study by Lin et al.,13 119 healthy teenagers engaged in an exhaustive exertion regimen consisting of 120 push-ups in five minutes. CK values in these subjects ranged from 55–174,260 with a mean of 36, 512. Sinert et al.14 observed average CK levels of 40,471 at admission for 35 patients with a discharge diagnosis of exercise-induced rhabdomyolysis in an urban tertiary care center study.
Prior studies on the TASER X26 showed no deleterious effects on breathing.7,10 In this study, there was a trend to a decrease in minute ventilation in the subjects with chest or back exposures (two devices), although it did not reach statistical significance. The subjects with three devices had lower chest to abdomen activation. These subjects did not show a trend to decreased minute ventilation. This may be because they had predominantly abdominal muscle activation rather than chest wall activation. This also clearly demonstrates that the diaphragm is not captured in this position.
The only change in ECG reflected some increased vagal tone after the discharge. There were no important rhythm changes. One subject had new non-specific inferior T wave changes as interpreted by the blinded cardiologist. The significance of these changes is not clear. This subject did not report any cardiac complaints, and had a normal troponin at 24 hours. These results are consistent with those of other authors.15,16 The echocardiography results showed no evidence of capture of the myocardium. In one subject, the location of the exposure and the heart rate would not be suggestive of capture. In the other subject, the rate was not a divisor of the pulse rate, suggesting that there was not capture. In addition, although the echocardiographer reported the heart rate increased within the first few seconds, the rate slowed more gradually after the exposure to the rate of 77. It was not an abrupt transition. Also, the initial heart rate (87) was measured in the minute before the exposure, not necessarily immediately before the exposure. It was clear to subjects when the exposure was about to take place, so his immediate initial heart rate may have been much higher than 87. So, even though capture cannot be excluded with certainty, it seems unlikely. In any event, the rate was not concerning.
Because the Shockwave device is similar to simultaneous exposures from the TASER X26, this data may be extrapolated to multiple simultaneous X26 exposures of similar duration. The Shockwave device is programmed to deliver the exposures such that the pulses do not overlap. While this would not be the case for multiple, simultaneous X26 exposures, the low duty cycle of the device would make overlap unlikely. At 19 pulses per second, there is 53 msec between each 0.1 msec pulse.
LIMITATIONS
This study has several limitations. First, the small number of subjects limits the ability to draw conclusions. Second, we were unable to determine sinus rhythm in two of the six subjects, although one had a heart rate that would not be worrisome for cardiac capture, and the other did not have a divisor of the device pulse rate and capture was not likely given the observations of the echocardiographer. Third, the probe positioning varied between the two-device groups and three-device group. This was intentional, determined by training, to reflect expected field deployments, but it makes some of the comparisons, particularly with regard to lactate and CK, difficult. For example, while it is well known that a majority of human skeletal muscles usually comprise a relative balance of slow twitch and fast twitch fibers (about 40 to 70% of one type versus the other), variations do, in general, exist between muscles with a predominately postural function (tending then to have higher proportions of slow twitch fibers) versus those with mainly phasic activities (which tend to have higher percentages of fast fibers).17 Significant variations in skeletal muscle slow-versus-fast muscle fiber type distributions between individuals can also exist, dependent upon gender, age, genetic, and health influences. Assuming the electrical activation of motor neuron axons by the relatively intense and spatially broad electric field discharges used in this study to be relatively non-selective with respect to axon size (as has been seen in some applications of electrical stimulation for therapeutic purposes), it is possible then that probe positioning and separations in our study could also have introduced additional variations in measures of muscle mass activation (lactate) and damage (CK) based upon underlying skeletal muscle fiber-dependent abilities to produce and maintain force.18 Lastly, the exposure duration in this study is short. The physiological changes with longer exposures may be different. Some changes may be expected to be more significant, such as with lactate and CK. Some, such as respiration, may actually show improvement with longer exposures. In prior studies, it was the experience of the authors that subjects often held their breath for the first 5–7 seconds due to a pain response and then began a more regular breathing pattern.
CONCLUSION
In our small sample size, five-second, simultaneous, multiple exposures to the TASER Shockwave device did not appear to have significant deleterious effects on human physiology, except for moderate increases in CK and elevations in lactate that are less than maximal exertion regimens, and possibly a trend towards decreased minute ventilation with large spread simultaneous exposures. Our study suggests that this device may have a reasonable risk/benefit ratio when used to protect an area from a threat. We recommend further study in this area to validate our results.
Footnotes
The authors would like to acknowledge the efforts of Andrew Hinz, Katie and Matt Carver, and Erik Lundin.
Supervising Section Editor: Eric D. Isaacs MD
Submission history: Submitted January 28, 2009; Revision Received April 14, 2009; Accepted September 14, 2009
Full text available through open access at http://escholarship.org/uc/uciem_westjem
Address for Correspondence: Jeffrey Ho, MD, Department of Emergency Medicine, Hennepin County Medical Center, 701 Park Avenue South, Minneapolis, MN 55415
Email: jeff.ho@hcmed.org
Conflicts of Interest: By the WestJEM article submission agreement, all authors are required to disclose all affiliations, funding sources, and financial or management relationships that could be perceived as potential sources of bias. TASER International provided partial funding for this study.
REFERENCES
1. Amnesty International Canada: Inappropriate and excessive use of TASERS. Amnesty International Canada. 2007
2. Nanthakumar K, Billingsley IM, Masse S, et al. Cardiac electrophysiological consequences of neuromuscular incapacitating device discharges. J Am Coll Cardiol. 2006;48:798–804. [PubMed]
3. Walter RJ, Dennis AJ, Valentino DJ, Margeta, et al. TASER X26 in swine produce potentially fatal ventricular arrhythmias. Acad Emerg Med. 2008;15:66–73. [PubMed]
4. Ho JD, Reardon RF, Dawes DM, et al. Ultrasound measurement of cardiac activity during conducted electrical weapon application in exercising adults. Ann Emerg Med. 2007;50:S108.
5. Ho JD, Dawes DM, Reardon RF, et al. Echocardiographic evaluation of human transcutaneous TASER application along the cardiac axis. Heart Rhythm. 2008;5:S348.
6. Jauchem JR, Cook MC, Beason CW. Blood factors of Sus scrofa following a series of three TASER exposures. Forensic Sci Int. 2008;175:166–70. [PubMed]
7. Ho JD, Dawes DM, Bultman LL, et al. Respiratory effect of prolonged electrical weapon application on human volunteers. Acad Emerg Med. 2007;14:197–201. [PubMed]
8. Enoka RM. Morphologic features and activation patterns of motor units. J Clin Neurophysiol.1995;12:538–59. [PubMed]
9. Ding J, Wexler A, Binder-MacLeod SA. A mathematical model that predicts the force-frequency relationship of human skeletal muscle. Muscle Nerve. 2002;26:477–85. [PubMed]
10. Vilke G, Sloane C, Bouton K, et al. Physiological effects of a conducted electrical weapon on human subjects. Ann Emerg Med. 2007;50:569–75. [PubMed]
11. Gass G, Rogers S, Mitchell R. Blood lactate concentration following maximum exercise in trained subjects. Brit J Sports Med. 1981;15:172–6. [PMC free article] [PubMed]
12. Ho JD, Miner JR, Lakireddy DR, et al. Cardiovascular and physiologic effects of conducted electrical weapon discharge in resting adults. Acad Emerg Med. 2006;13:589–95. [PubMed]
13. Lin AC, Lin CM, Wang TL, et al. Rhabdomyolysis in 119 students after repetitive exercise. Br J Sports Med. 2005;39:e3. [PMC free article] [PubMed]
14. Sinert R, Kohl L, Reinone T, et al. Exercise-induced rhabdomyolysis. Ann Emerg Med.1994;23:1301–6. [PubMed]
15. Vilke G, Sloane C, Levine S, et al. Twelve-lead electrocardiogram monitoring of subjects before and after voluntary exposure to the TASER X26. Am J Emerg Med. 2008;26:1–4. [PubMed]
16. Levine S, Sloane C, Chan T, et al. Cardiac monitoring of human subjects exposed to the TASER. J Emerg Med. 2007;33:113–7. [PubMed]
17. Johnson MA, Polfar J, Weightman D, et al. Data on the distribution of fibre types in thirty-six human muscles: An autopsy study. J Neurol Sci. 1973;18:111–29. [PubMed]
18. Gregory CM, Bickel CS. Recruitment patterns in human skeletal muscle during electrical stimulation. Phys Ther. 2005;85:358–64. [PubMed]