Author | Affiliation |
---|---|
Jeffery S. Shafer, MD, EMTP | Washington University School of Medicine, Division of Emergency Department |
Rosanne S. Naunheim, MD | Washington University School of Medicine, Division of Emergency Department |
ABSTRACT
Spinal immobilization is one of the most commonly performed pre-hospital procedures. Little research has been done on the movement of the neck during immobilization and extrication. In this study we used a sophisticated infrared six-camera motion-capture system (Motion Analysis Corporation, Santa Rosa, CA), to study the motion of the neck and head during extrication. A mock automobile was constructed to scale, and volunteer patients, with infrared markers on bony prominences, were extricated by experienced paramedics. We found in this pilot study that allowing an individual to exit the car under his own volition with cervical collar in place may result in the least amount of motion of the cervical spine. Further research should be conducted to verify these findings. In addition, this system could be utilized to study a variety of methods of extrication from automobile accidents.
INTRODUCTION
It is estimated that 3–25% of spinal cord injuries may be significantly worsened during transport or early treatment, and therefore are preventable.1,2 Because of this concern for subsequent injury, full spinal immobilization remains the standard of care for Emergency Medical Services (EMS) throughout much of the United States.1 Currently, full spinal immobilization, as recommended by the American College of Surgeons, consists of application of a cervical collar (CC), immobilization on a long backboard, and the addition of lateral immobilizing devices.3,1 The Kendrick Extrication Device (KED) is also available as an effective adjunct to spinal immobilization.4,5,6
However, full spinal immobilization is not without risk. It has been associated with a multitude of complications, including airway compromise, aspiration, increased intracranial pressure, cutaneous pressure ulcers, iatrogenic pain, combativeness of intoxicated patients and increased cost and time of extrication.7–22 And backboards place most patients in a position of relative cervical extension.18,23,24 Removal of patients from a spine board is also problematic with studies showing that “log rolling” a patient results in significant motion of the thoracolumbar spine.25 Given that the majority of patients who are trauma packaged will have no spinal injury, efforts are underway to identify those whom EMS personnel may safely forego spinal immobilization.26–29 While pre-hospital cervical spine clearance may prove successful, not all patients will be candidates, possibly due to serious or distracting injury, intoxication, or neck pain. Even if EMS medical directors were to adopt pre-hospital cervical spine clearance protocols, they would require considerable effort to institute and maintain.
While immobilization is problematic in itself, a broader question that must be answered is whether the act of immobilizing the spine results in movement of vertebral segments. Spinal motion has been studied with cadavers, using photogrammetry (analysis of multiple photographs recreating a three-dimensional picture by sterotaxis)30,31 and via radiographic analysis of cervical motion.4,5
Previously, no one had the ability to examine spinal movement during immobilization and actual extrication from an automobile. Roozmon32,33 had suggested a more comprehensive motion-capture system as the best method for further study of motion in the cervical spine. This system has been used to study kinematics and cervical motion.34,35 Pearcy36 demonstrated that skin markers reflect the underlying bony structure if they are placed over relatively fixed points in the skin; ie., sternum, acromion and zygoma. (In a previous study, the displacement error in finding bony landmarks has been estimated at less than one degree.)37 This study36 also defined the orthogonal base vector system for two rotating bodies, which has become the standard for motion-capture systems. By using a group of markers to define a plane, the relative motion of the head as compared to the torso can be determined mathematically. This involves the absolute angles of the orthogonal vectors in each frame, which are determined trigonometrically with respect to a fixed calibration frame. Next, a transformation matrix rotates the coordinate systems to the absolute reference frame by using Euler’s angles to translate from one coordinate system to another.
Our goal was to conduct a pilot study using an infrared video motion-capture system to examine, for the first time, extrication from a mock automobile. We will determine feasibility of further studies with the motion-capture equipment and provide preliminary data.
METHODS
The study was approved by the Washington University Institutional Review Board, and written informed consent was obtained from all parties.
Using as our model a 2001 Toyota Corolla that had significant damage to the interior compartment, with significant dash intrusion and steering wheel deformity, we constructed a mockup to scale, including ground height, floorboard space, dash, center console, steering wheel, ceiling, and doors. We included all deformity rendered by the high-speed accident. The actual Toyota seats were removed and placed in the mock vehicle. To allow visualization of markers by the motion analysis system, direct line of sight with two of six cameras had to be established and maintained at all times. Therefore, we removed the seat back cushions and replaced them with plexiglass. The frame of the vehicle was constructed from ½” PVC conduit and a bent-wire frame.
The Motion Analysis Corporation (MAC) six-camera motion-capture system (Santa Rosa, CA) was used to track 0.5 inch reflective markers on the head (forehead, crown, zygomas), C7, and the trunk (acromion, humerus, clavicle, sternum, anterior superior iliac spine, and greater trochanters bilaterally). This allowed the identification of planes defining the head and torso. Calibration of the system involved measuring deviations from known distances between fixed markers and deviations from known angles as measured by the six cameras, using a triangulation system with the EVa Real-Time Software (EVaRT) (MAC, Santa Rosa, CA). We recorded the position of each marker (calibrated accuracy to 0.5mm) using EVaRT at a frame rate of 60/sec. Standard analysis programs (Excel) allowed the calculation of the change of angle between the head and torso. Starting position of the subject was in the driver’s seat of the mock automobile.
We recruited three paramedics, each with more than five years EMS experience. One paramedic, acting as the driver, was extricated by the other two using each of four techniques:
-
The “driver” was allowed to exit the vehicle on his/her own volition and lie on a backboard.
-
The “driver” was allowed to exit the vehicle on his/her own volition with a CC in place and lie on a backboard.
-
The “driver” was extricated head first via standard technique by the remaining two paramedics with a CC alone.29,30 (Standard technique involves turning the driver so that the legs are in the passenger’s seat, allowing the driver to lie back and raising the right hip so a long board can be placed under the hip. A second paramedic who enters the front seat passenger’s door helps slide the “driver” up on to the board.)
-
The “driver” was extricated head first via standard technique by the remaining two paramedics with a CC and KED.
RESULTS
We were able to calculate the absolute angle of movement of the cervical spine using extrapolated lines connecting the head (forehead, crown, zygomas), C7, and the trunk (acromion, humerus, clavicle, sternum, anterior superior iliac spine, and greater trochanters bilaterally) which created planes of the head and the torso, respectively.
Ultimately, we documented the least movement of the cervical spine in subjects who had a cervical collar applied and were allowed to simply get out of the car and lie down on a stretcher. [mean change 1.4 ± 4.0 deg, and peak change 6.8 ± 1.8 deg]. See Table 1. Extricating the driver/subject head-first by standard technique to a long spine board was associated with significant cervical spine motion, both with the collar alone [mean change 1.0 ± 4.5 deg, and peak change 26.6 ± 14.2 deg] and even with a cervical collar and KED [mean change 2.0 ± 2.3 deg, and peak change 31.1 ± 17.6 deg]. See Table 2.
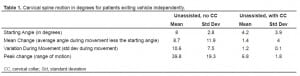
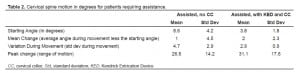
DISCUSSION
The American Association of Neurological Surgeons and the Congress of Neurological Surgeons recognized in 2002 that insufficient evidence exists to support treatment standards or guidelines with respect to pre-hospital spinal immobilization.1 However, they acknowledge that it is unlikely that all trauma patients require full spinal immobilization.1 Some patients, such as those with neurologic deficits or altered mental status clearly will require full immobilization for transport and protection of the spine. However, full immobilization of patients with isolated neck or back pain may result in more manipulation of the spine than simply allowing those patients to move themselves.
The National Association of EMS Physicians Standards and Clinical Practice Committee26states that patients without altered mental status, intoxication, neck or back pain/tenderness, or distracting injury may forego spinal immobilization. Of two recent studies, only 48 of 13,652 patients with spinal injuries were missed by application of this pre-hospital criteria.28,29 No patient suffered an adverse outcome. At least one retrospective study suggests that ambulatory trauma patients have little/no risk of thoracolumbar fractures.34 We may never have the capability to discern which movements result in worsening injury, since this is dependent on the type of injury and the specific individual. The best course of action may be to identify those at high risk for possible injuries through clinical criteria and treat them with the method involving the least spinal movement.
LIMITATIONS
We noted several limitations of the motion capture system. Flexion/extension of the cervical spine may not be analyzed correctly if a line drawn through the frontal plane of the head and a second line drawn through the acromia representing the torso both flex forward, causing the relative motion to be zero. We remedied this by creating a three-point plane of the head and a second one for the torso. We also placed a marker on C7 (with a small portion of the CC removed); however, the marker was still only intermittently visible during the extrication process. Ultimately video was needed to exclude the presence of any flexion/extension. We also needed video to exclude the presence of isolated shoulder movement. These errors may be remedied in future studies by placement of additional markers. In addition, the MAC system was unable to provide sufficient data to evaluate movement of the thoracolumbar spine. (Hardware not requiring line of sight for location of markers will prove superior in the future, if markers are small). Neither were we able to obtain sufficient pelvic data from the markers located over the greater trochanters and anterior superior iliac spines to elucidate any movement of the thoracolumbar spine. This was particularly true when the KED was placed.
Other limitations include the use of a mock automobile and our choice of subjects. We involved only healthy, cooperative, EMS-educated personnel, whose depth of medical knowledge was another drawback.
This study was designed to serve as a pilot study. No changes in current treatment protocols should be made based on it alone. Our research was limited by a lack of power to make such determinations.
A more definitive, appropriately powered study should be conducted to demonstrate if allowing ambulatory patients to leave the vehicle independently with CC alone ± an adjunctive device would be superior to standard immobilization on a backboard. It will be necessary to study a larger number of patients. We further hope that, in the future, we may use this technology to study a variety of extrication techniques for those patients who do require full spinal immobilization.
CONCLUSION
In those ambulatory subjects who do not complain of back pain, the least motion of the cervical spine may occur when the subject is allowed to exit the car in a c-collar without backboard immobilization. This may have implications for decreasing extrication time in the pre-hospital setting and reducing complications of long spine board use.
Footnotes
Supervising Section Editor: Christopher A. Kahn, MD, MPH
Submission history: Submitted May 29, 2008; Revision Received September 29, 2008; Accepted November 02, 2008
Full text available through open access at http://escholarship.org/uc/uciem_westjem
Address for Correspondence: Jeffery S. Shafer, MD, EMTP, Washington University School of Medicine, Division of Emergency Medicine, 660 S. Euclid Ave, Campus Box 8072, St. Louis, MO 63110
Email: shaferj@wusm.wustl.edu.
Conflicts of Interest: By the WestJEM article submission agreement, all authors are required to disclose all affiliations, funding sources, and financial or management relationships that could be perceived as potential sources of bias. The authors disclosed none.
REFERENCES
1. Hadley MN, Walters BC, Grabb PA, Oyesiku NM, Przybylski GJ, Resnick DK, Ryken TC, et al. Guidelines for the management of acute cervical spine and spinal cord injuries. Clin Neurosurg. 2002;49:407–98. [PubMed]
2. Toscano J. Prevention of neurological deterioration before admission to a spinal cord injury unit. Paraplegia. 1998;26:143–150. [PubMed]
3. American College of Surgeons Committee on Trauma. Advanced Trauma Life Support.7th ed. Chicago, IL: American College of Surgeons; 2004. p. 187.
4. Howell JM, Burrow R, Dumontier C, Hillyard A. A practical radiographic comparison of short board technique and Kendrick Extrication Device. Ann Emerg Med. 1989;18:943–6.[PubMed]
5. Graziano AF, Scheidel EA, Cline JR, Baer LJ. A radiographic comparison of pre-hospital cervical immobilization methods. Ann Emerg Med. 1987;16:1127–1131. [PubMed]
6. Kwan I, Bunn F. Effects of prehospital spinal immobilization: a systematic review of randomized trials on healthy subjects. Prehosp Disast Med. 2005;20:47–53.
7. Bauer D, Kowalski R. Effect of spinal immobilization devices on pulmonary function in the healthy, nonsmoking man. Ann Emerg Med. 1988;17:915–8. [PubMed]
8. Schafermeyer RW, Ribbeck BM, Gaskins J, Thomason S, Harlan M, Attkisson A. Respiratory effects of spinal immobilization in children. Ann Emerg Med. 1991;20:1017–9. [PubMed]
9. Jedlicka DS. A comparison of the effects of two methods of spinal immobilization on respiratory effort in the older adult. Diss Abstra Int. 1999;58-05B:2354.
10. Totten VY, Sugarman DB. Respiratory effects of spinal immobilization. Prehosp Emerg Care. 1999;3:347–352. [PubMed]
11. Hamilton RS, Pons PT. The efficacy and comfort of full-body vacuum splints for cervical-spine immobilization. J Emerg Med. 1996;14:553–559. [PubMed]
12. Chan D, Goldberg RM, Mason J, Chan L. Backboard versus mattress splint immobilization: A comparison of symptoms generated. J Emerg Med. 1996;14:293–298.[PubMed]
13. Cordell WH, Hollingsworth JC, Olinger ML, Stroman SJ, Nelson DR. Pain and tissue-interface pressures during spine-board immobilization. Ann Emerg Med. 1995;26:31–36.[PubMed]
14. Lerner EB, Billittier AJ, 4th, Moscati RM. The effects of neutral positioning with and without padding on spinal immobilizatoi nof healthy subjects. Prehosp Emerg Care.1998;2:112–116. [PubMed]
15. Delbridge TR, Auble TE, Garrison HG, Menegazzi JJ. Discomfort in healthy volunteers immobilized on wooden backboards and vacuum mattress splints. Prehosp Disast Med.1993;8(3 Supplement):S63.
16. Barney R, Cordell W. Pain associated with immobilization on rigid spine boards. Ann Emerg Med. 1989;18:918.
17. Johnson DR, Hauswald M, Stockhoff C. Comparison of a vacuum splint device to a rigid backboard for spinal immobilization. Am J Emerg Med. 1996;14:369–72. [PubMed]
18. Walton R, DeSalvo JF, Ernst AA, Shahane A. Padded vs. unpadded spine board for cervical spine immobilization. Acad Emerg Med. 1995;2:725–8. [PubMed]
19. Chan D, Goldberg R, Tascone A, Harmon S, Chan L. The effect of spinal immobilization on healthy volunteers. Ann Emerg Med. 1994;23(1):48–51. [PubMed]
20. Hauswald M, Hsu M, Stockoff C. Maximizing comfort and minimizing ischemia: a comparison of four methods of spinal immobilization. Prehosp Emerg Care. 2000;4:250–2. [PubMed]
21. Linares HA, Mawson AR, Suarez E, Biundo JJ. Association between pressure sores and immobilization in the immediate post-injury period. Orthopedics. 1987;10:573.
22. Mawson AR, Biundo JJ, Jr, Neville P, Linares HA, Winchester Y, Lopez A. Risk factors for early occurring pressure ulcers following spinal cord injury. Am J Phys Med & Rehabil.1988;67:123–127. [PubMed]
23. De Lorenzo RA, Olson JE, Boska M, Johnston R, Hamilton GC, Augustine J, Barton R. Optimal positioning for cervical immobilization. Ann Emerg Med. 1996;28:301–8.[PubMed]
24. Schriger DL, Larmon B, LeGassick T, Blinman T. Spinal immobilization on a flat backboard: does it result in neutral position of the cervical spine? Ann Emerg Med.1991;20:878–81. [PubMed]
25. Swartz E, Nowak J, Shirley C, Decoster L. A comparison of head movement during back boarding by motorized spine-board and log-roll techniques. J Athl Training.2005;40:162–168.
26. Domeier RM. Indications for prehospital spinal immobilization. National Association of EMS Physicians Standards and Clinical Practice Committee. Prehosp Emerg Care.1999;3:251–3. [PubMed]
27. Domeier RM, Swor RA, Evans RW, Hancock JB, Fales W, Krohmer J, Frederiksen SM, et al. Multicenter prospective validation of prehospital clinical spinal clearance criteria. J Trauma. 2002;53:744–50. [PubMed]
28. Domeier RM, Frederiksen SM, Welch K. Prospective performance assessment of an out-of-hospital protocol for selective spine immobilization using clinical spine clearance criteria. Ann Emerg Med. 2005;46:123–31. [PubMed]
29. Burton JH, Harmon NR, Dunn MG, Bradshaw JR. EMS provider findings and interventions with a statewide EMS spine-assessment protocol. Prehosp Emerg Care.2005;9:303–9. [PubMed]
30. Yoganandan N, Pintar F, Gennarelli T. High-speed 3-D kinematics from whole-body lateral impact sled tests. Biomed Sci Instrum. 2007;43:40–5. [PubMed]
31. Yoganandan N, Pintar F, Reinartz J, Cusick JF, Maiman DJ, Sances A. Continuous motion analysis of the head-neck complex under impact. J Spinal Disord. 1994;7:420–8.[PubMed]
32. Roozmon P, Gracovetsky SA, Gouw GJ, Newman N. Examining motion in the cervical spine. I: Imaging systems and measurement techniques. J Biomed Eng. 1993;15:5–12.[PubMed]
33. Roozmon P, Gracovetsky SA, Gouw GJ, Newman N. Examining motion in the cervical spine. II: Characterization of coupled joint motion using an opto-electronic device to track skin markers. J Biomed Eng. 1993;15:13–22. [PubMed]
34. Nigg BM. Measuring techniques. In: Nigg BM, Herzog W, editors. Biomechanics of the Musculo-Skeletal System. New York, NY: Wiley; 1994. pp. 268–78.
35. Richards J. The measurement of human motion: a comparison of commercially available systems. Hum Mov Sci. 1999;18:589–562.
36. Pearcy MJ. Measurement of human back movements in three dimensions by opto-electronic devices. Clin Biomech. 1987;20:943–9.
37. Plamondon A, Gagnon M. Evaluation of Euler’s angles with a least squares method for the study of lumbar spine motion. J Biomed Eng. 1990;12:143–9. [PubMed]
38. Tamir E, Anekstein Y, Mirovsky Y, Heim M, Dudkiewicz I. Thoracic and lumbar spine radiographs for walking trauma patients–is it necessary? J Emerg Med. 2006;31:403–5.[PubMed]