Author | Affiliation |
---|---|
Jesse G.A Jones, MD | Los Angeles County–USC Medical Center, USC Keck School of Medicine, Department of Radiology, Los Angeles, California |
Christopher N. Mills, MD, MPH | Inova Fairfax Hospital, Department of Emergency Medicine, Falls Church, Virginia |
Monique A. Mogensen, MD | Los Angeles County–USC Medical Center, USC Keck School of Medicine, Department of Radiology, Los Angeles, California |
Christoph I. Lee, MD | UCLA David Geffen School of Medicine, Departments of Internal Medicine and Radiology, Los Angeles, California |
ABSTRACT
Introduction:
Medical imaging now accounts for most of the US population’s exposure to ionizing radiation. A substantial proportion of this medical imaging is ordered in the emergency setting. We aim to provide a general overview of radiation dose from medical imaging with a focus on computed tomography, as well as a literature review of recent efforts to decrease unnecessary radiation exposure to patients in the emergency department setting.
Methods:
We conducted a literature review through calendar year 2010 for all published articles pertaining to the emergency department and radiation exposure.
Results:
The benefits of imaging usually outweigh the risks of eventual radiation-induced cancer in most clinical scenarios encountered by emergency physicians. However, our literature review identified 3 specific clinical situations in the general adult population in which the lifetime risks of cancer may outweigh the benefits to the patient: rule out pulmonary embolism, flank pain, and recurrent abdominal pain in inflammatory bowel disease. For these specific clinical scenarios, a physician-patient discussion about such risks and benefits may be warranted.
Conclusion:
Emergency physicians, now at the front line of patients’ exposure to ionizing radiation, should have a general understanding of the magnitude of radiation dose from advanced medical imaging procedures and their associated risks. Future areas of research should include the development of protocols and guidelines that limit unnecessary patient radiation exposure.
INTRODUCTION
Over the last 2 decades, a dramatic rise in the use of diagnostic computed tomography (CT) has led to concerns about increased cancer risks from cumulative exposure to ionizing radiation.1,2 Debate continues regarding the lifetime risk of fatal cancer imparted by any single imaging study or series of studies. However, several prominent regulatory and scientific societies state that no radiation dose is without carcinogenic risk.3
Although the American College of Radiology (ACR) and Society for Pediatric Radiology have addressed concerns regarding radiation dose with campaigns such as “Image Gently” and “Image Wisely,” recent media reports of unnecessary exposure from CT have garnered national attention. One recent report found that 206 patients undergoing emergent “rule out stroke” CT protocols at a single hospital received up to 8 times the standard radiation dose for perfusion CT, which amounts to the equivalent of 200 noncontrast head CT.4 Such errors have prompted investigation by the US Food and Drug Administration (FDA) and calls for a framework to monitor radiology examination indications, dose delivery, and imaging history.5
As the volume of medical imaging obtained in the emergency department (ED) has increased over the last 2 decades, emergency physicians are now at the forefront of determining patients’ exposure to ionizing radiation.6 Indeed, from 1995 to 2007 the number of ED visits that included a CT examination has burgeoned from 2.7 million to 16.3 million.7 Emergency physicians must be aware of risks inherent to ionizing radiation, available alternative imaging modalities, and appropriateness of each study. Currently, there are no review articles in the emergency medicine literature focused specifically on radiation dose in the ED setting. This article provides a general review of the current literature regarding radiation dose in the ED, highlighting recent efforts while also identifying areas for future research aimed at decreasing unnecessary radiation exposure in the emergency setting.
METHODS
We conducted a literature search for all journal publications regarding radiation dose from medical imaging in the ED setting in MEDLINE through calendar year 2010. Specifically, we performed a literature search for all articles with no limitations on date, type of journal article, or language by using the keywords “emergency medicine,” “radiation dose,” and several variations and combinations of the keywords. The first and last author independently reviewed 90 preliminary articles for relevance to the ED and radiation exposure from medical imaging. We included all articles pertaining to the level of radiation dose experienced by patients in the ED, the education or awareness of emergency physicians regarding radiation dose, and initiatives to reduce radiation exposure in the ED. We then reviewed all related citations for these preliminary articles that we deemed relevant, including all citations from the bibliography section of the selected articles.
To provide information specific to emergency physicians, we start with a brief, general overview of radiation dose terminology and then organize the results of our literature review into 3 commonly encountered clinical presentations for which medical imaging plays a major role in making the diagnosis in the ED: pulmonary embolism, renal colic, and recurrent abdominal pain in patients with inflammatory bowel disease. For each of these topics, we refer to ACR appropriateness criteria for medical imaging.8 We chose to exclude articles regarding radiation dose in trauma patients for whom rapid imaging likely outweigh the risks of radiation in most cases. We also excluded articles for the special cases of children and pregnant females for which discussion of radiation dose is beyond the scope of this general primer and would be more appropriately discussed in a separate article. Thus, for the purposes of our article we provide a literature review of specific clinical scenarios for adult, nonpregnant patients for whom action can be taken to decrease radiation exposure by avoiding equivocal or marginally indicated CT.
RESULTS
General Overview of Radiation Dose
Radiologic procedures that produce ionizing radiation include CT, plain radiography, nuclear medicine, and fluoroscopy. The largest component of imaging-based radiation stems from CT, which is the focus of our article. Ionizing radiation causes damage at the cellular level via free radical formation, eventually leading to DNA mutation or cell death.9,10 Cellular mutations may eventually lead to radiation-induced cancers including leukemia, myeloma, or cancer of the thyroid, breast, lung, bone, and skin.11
While the magnitude of cancer induction from any individual exposure to ionizing radiation cannot be measured exactly, the most widely accepted theoretical dose-response model is the linear no-threshold model.12–15 This model is an extrapolation of atomic bomb survivor data from Hiroshima and Nagasaki and is the most conservative, assuming even the smallest exposure to ionizing radiation has the potential to induce future cancer.16,17 The US National Academy of Sciences Biological Effects of Ionizing Radiation (BEIR) and the US National Council on Radiological Protection and Measurements both suggest the use of this model for estimating risks of ionizing radiation.11,18
Several measurements are available for quantifying radiation dose. The quantity of ionizing radiation is measured in Gray (Gy) and is analogous to the older unit called a rad (radiation absorbed dose). Each radioactive particle—alpha, beta, and gamma rays (gamma is used in radiographs)—has a unique ionizing effect on biological tissue. This “equivalent dose” of gamma rays is expressed as the sievert (Sv) or rem (radiation equivalent in man). The conversion for radiographs is 1:1 such that 1 Gy of radiograph = 1 Sv (or 1 rad = 1 rem). Alpha and beta particles, emitted from a nuclear reactor or radiopharmaceutical, produce different effective doses and are beyond the scope of this review.
The term effective dose is widely used in the medical community and represents the weighted average of doses absorbed by irradiated organs, therefore reflecting the equivalent whole-body dose that would result in an equivalent risk from a nonuniform radiation source. It provides an estimate of a patient’s risk of harm from any radiologic procedure, including all possible future cancers and hereditary effects. The effective dose allows for comparison across different imaging modalities and distributions across the body. However, the biological effect of radiation exposure varies substantially with age. It is most pronounced for young patients, whose organs are in closer proximity and whose cells are undergoing constant mitosis.
Moreover, the effective dose for radiologic studies can be considered in the context of normal annual background radiation for the general public. At approximately 3 mSv per year, the average background radiation from radon, cosmic rays, and other sources is not considered to be a significant cancer risk. For common radiologic procedures with an effective dose of 10 mSv (approximately equivalent to 1 CT of the chest, abdomen, or pelvis), the 2006 BEIR VII lifetime attributable cancer risk model predicts that 1 in 1,000 persons will develop cancer due to such an exposure.18,19 Cumulative effective dose greater than 100 mSv from repeated exposures shows even more convincing evidence for increased cancer risk.1,20,21 A cumulative exposure of 1 Sv (1,000 mSv) confers a 4% to 5% increased relative risk of fatal cancer according to the International Commission on Radiological Protection.22 As discussed above, relative cancer risk varies with age, and, therefore, these estimates must be adjusted for younger and older patients accordingly.
We provide a table with average effective doses for common radiologic procedures ordered in the emergency setting (Table). This table is adapted from a number of recent reports in the medical literature and provides effective doses for adults.23–28 A recent study suggests that average effective doses may differ by up to 10-fold depending on specific imaging protocols and equipment settings.23Nevertheless, these doses allow for a general estimate of the risks associated with each type of study and can be used in helping to determine the risks in relation to the benefits of emergent radiologic procedures. Chest radiograph equivalents are also provided in the Table, which may be helpful in any patient-physician discussion regarding radiation dose from medical imaging.
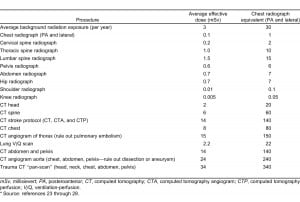
Rule Out Pulmonary Embolism
Patients with suspected acute pulmonary embolism (PE) require rapid and accurate diagnosis to initiate anticoagulation. Workups for PE should begin with clinical risk stratification using standardized criteria. The Wells score and revised Geneva score are 2 commonly used prediction rules that can divide cases into high or low pretest probability of PE by risk factors and biomarkers.29–33 False-positive D-dimer test results in low-risk patients are common, however, and thus can lead to unnecessary CT if D-dimer testing is used for extremely low-risk populations. This problem prompted development of the PE rule-out criteria (PERC), which selects a population with low enough risk to not warrant D-dimer testing. PERC allows for risk stratification in which a negative score correlates with an acceptable, lower than 2% outcome rate of PE.34–36
Posteroanterior and lateral chest radiographs serve as a useful screening tool that may reveal an alternative cause for pleuritic chest pain.37,38 If advanced imaging is necessary, CT pulmonary angiography (CTPA) of the chest has overtaken ventilation-perfusion (V/Q) scans as the modality of choice for suspected PE.39,40 The Prospective Investigation of Pulmonary Embolism Diagnosis II trial demonstrated that CTPA was more sensitive for detecting PE than V/Q scans, though the 2 modalities had similar positive predictive values.40 The CTPA technique has the added advantage of being able to identify alternative diagnoses such as aortic pathology, pneumothorax, or pericardial effusion.41
While CTPA is the imaging modality of choice, the total effective dose from CTPA is about 5 times greater than that from V/Q scans.25,42–44 Ventilation-perfusion scanning remains a valuable diagnostic modality in patients with contrast allergies, renal insufficiency, excessive obesity, and claustrophobia.45 Unfortunately, V/Q scans are frequently indeterminate such that further diagnostic evaluation is often required. Indeterminate V/Q scans can be minimized by selecting patients who are young and have normal chest radiographic findings. Moreover, while fetal radiation is comparable between V/Q and CTPA, there is much less radiation exposure to radiosensitive breast tissue in pregnant females from V/Q scans than from CTPA.2 Thus, V/Q scanning remains the primary modality for imaging female patients of child-bearing age with suspected PE and normal chest radiographic findings. Also, the dose of radiopharmaceutical in V/Q scans can be decreased by at least a factor of 3 with longer acquisition times in order to reduce radiation in pregnancy.46
Renal Colic
Urolithiasis is a common condition that can lead to renal dysfunction if concomitant hydronephrosis is left untreated.47 Imaging plays a role in the evaluation of suspected urolithiasis by demonstrating a stone’s size, location, and effect on renal anatomy. Imaging can confirm the diagnosis and distinguish patients who need urologic intervention from those who do not. Moreover, an appropriate imaging study can also rule out more sinister etiologies of flank pain, such as renal cancer or nonurinary disorders such as appendicitis, diverticulitis, ovarian pathology, and abdominal aortic aneurysm (AAA).48
CT is the modality of choice for evaluating adult patients with signs and symptoms of renal colic presenting for the first time.49 A noncontrast CT of the abdomen and pelvis can quickly identify 95% of stones and rule out diagnoses such as appendicitis and AAA. Radiologists can also adjust certain parameters to reduce the effective radiation dose.50,51 These lower-dose protocols have effective doses as small as 1.5 mSv while maintaining high sensitivity and specificity.52–54 Recent studies have shown that low-dose CT stone protocols have the same sensitivity as usual CT for stones 3 mm or greater in size.51,55
Some studies have suggested ultrasound (US) and magnetic resonance imaging (MRI) as alternatives to CT for renal colic.56 However, institution-specific expertise and availability are limiting factors with both modalities. US of the urinary tract is highly operator-dependent, with sensitivity of US for acute flank pain reported at 61% to 93% and largely limited by poor visualization of the ureters.57–59While plain radiography with US would greatly decrease radiation dose by an order of magnitude, sensitivity is lower than for CT.60–62 This is why US leads to many equivocal studies for which CT may ultimately be necessary.63 Finally, although MRI can identify ureteral obstruction, its limited availability and suboptimal power to identify the cause and exact location limits its use.64
Up to 50% of stone formers will suffer a recurrence within 10 years2 and may undergo many CT.65,66Katz et al66 reported on the use of CT stone protocols for 4,562 patients during a 6-year period. They found that a single stone protocol CT imparted an effective dose between 6.5 and 8.5 mSv, with 4% of patients obtaining 3 or more CT examinations with a cumulative radiation dose between 10.5 and 153.7 mSv. In another study of 262 ED patients with renal colic who underwent CT, 92 patients had 3 or more studies within 10 months.67 Repeated CT of chronic stone formers, compared to patients presenting with renal colic for the first time, has been shown to rarely change the diagnosis or treatment plan.68
This population, thus, presents a dilemma to the emergency physician, who must weigh the risks of additional radiation from yet another CT against the risks of a missed alternative diagnosis or impassable stone. Combining US and kidney ureter bladder radiograph has been suggested as a first-line strategy for chronic stone formers with high pretest probability and low risk of other diagnoses.66 Others feel that a patient with renal colic symptoms and a history of stones with a US finding negative for hydronephrosis or AAA can be discharged from the ED with putative treatment for uncomplicated urolithiasis.
Recurrent Abdominal Pain in Inflammatory Bowel Disease
Abdominal pain is the most common chief complaint in the ED associated with CT.7 Diffuse nonspecific abdominal pain results in fewer positive CT findings than localized pain, and may benefit from a period of observation.69 However, no imaging substitute can effectively rule out a disease process with the sensitivity of CT, as evidenced by a 32% rate of positive findings in clinically ambiguous cases.69,70 In light of this, we advocate focusing dose-reduction strategies for patients with recurrent abdominal pain who are likely to undergo repeated CT, such as patients with inflammatory bowel disease (IBD).
IBD incidence peaks between 15 and 25 years of age, subjecting patients to a long period of remaining lifespan in which to manifest radiation-induced carcinogenesis.71 Moreover, IBD itself predisposes patients to gastrointestinal, liver, and biliary tract tumors.72 Recurrent flareups and complications such as strictures, fistulae, and abscesses lead patients to seek emergency care, with a high likelihood for obtaining repeated scans. CT utilization for such IBD complications has grown at a faster rate than other indications among the general population.73 A study of 409 patients with Crohn disease found that 15% received a high cumulative effective dose (>75 mSv), a level of exposure that has been reported to increase cancer mortality by as much as 7.3%.74
One alternative to CT for the IBD populations is US.75 US has proved effective in detecting features of active IBD in nonobese children, such as thickened bowel walls, hypoactive peristalsis, and increased mesenteric blood flow by Doppler.76–78 Bowel wall thickness greater than 2.5 mm in the terminal ileum or greater than 3 mm in the colon predicts active disease with 93% specificity.79 Although 1 study suggested that US and CT had comparable diagnostic accuracy in identifying fistulae and abscesses in patients with IBD,80 US in the setting of IBD remains highly operator-dependent, and sensitivity is variable between studies.81 Small-bowel obstruction, a common complication of Crohn disease, is best seen on CT rather than US.
MRI has gained increasing popularity in IBD evaluation. Active disease demonstrates thickened bowel that is enhanced on MRI with intravenous contrast.82 Compared to the gold standard of endoscopy or open surgery, these findings are more sensitive (92%) and specific (75%) than those of CT.83 MRI can identify abscesses and fistulas as well.84 Although availability and cost may limit use of MRI, its radiation-sparing effects are appealing.85 Factors correlated with greatest risk for repeated imaging in patients with IBD include early age of onset, corticosteroid therapy, and need for multiple surgeries. Griffey and Sodickson86 found that ED patients at 1 institution who underwent multiple CT had a mean cumulative radiation dose of 91 mSv and a lifetime attributable cancer risk of 1 in 110. These patients stand to gain the most benefit from choosing US or MRI over CT as the first line of imaging.
DISCUSSION
We have provided one of the first general overviews regarding radiation dose for the emergency medicine community and, to our knowledge, the first literature review regarding efforts to decrease unnecessary radiation exposure in the ED setting. It is obvious that CT imaging has had a transformative effect on emergency care by dramatically improving the speed and accuracy of diagnosis, thereby facilitating patient treatment and disposition. The explosion in CT utilization over the last 2 decades, however, has raised concerns about the deleterious effects of cumulative ionizing radiation. If current models of the carcinogenic risk imparted by low-dose radiation prove to be correct, CT may be contributing to a growing public health problem of radiation-induced cancers at the population level. Since ED physicians are ordering about one third of all CT studies in the United States, they must increasingly be knowledgeable about radiation dose and future radiation-induced cancer risks in their decision-making process, and possibly discuss these risks with their patients in cases for which the immediate benefits may not outweigh future risks.87
On the basis of our literature review, we can conclude that there are 3 specific clinical scenarios among adults for which the ED physician should consider the risks of ionizing radiation from CT before ordering such studies, and for which alternative imaging modalities may be appropriate. These include ruling out PE, renal colic, and recurrent abdominal pain. While the available literature focuses on possible avenues for decreasing radiation exposure in these specific situations, there is currently a paucity of rigorous and reliable protocols for ED physicians to follow in order to prevent unnecessary radiation exposure.
The most researched scenario for decreasing radiation dose to patients in the ED setting is the case of ruling out PE. A current review of the literature yields several steps that may help prevent unnecessary patient radiation exposure. First, standardized criteria for risk stratification should be used (eg, PERC rule or Wells score) for all cases. Second, an initial chest radiograph may reveal an alternative diagnosis and thus prevent the need for CTPA. Additionally, given its lower effective dose to the breasts, V/Q scanning should remain the primary modality for ruling out PE in pregnant patients and young women.
In recent years, abdominal pain has become the leading indication for CT in the ED, surpassing trauma and neurologic complaints.7 Unlike the case for PE, no established algorithms are in place to decrease CT studies among ED patients. Our literature review suggests that imaging abdominal pain varies with institutional preference, although centers with an interest in reducing radiation dose have developed low-dose CT protocols and the use of US as a screening tool for specific patient populations. Emerging studies suggest that low-dose CT protocols for renal colic may represent a compromise between risk of radiation and benefit of confirming the diagnosis of urolithiasis and possess equivalent sensitivity to standard CT.88,89
More recently, chronic abdominal pain and IBD populations have been singled out as being at increased risk of radiation-induced cancer. Some experts have suggested that US be the initial study for patients with repeated IBD to assess for disease exacerbation. A diagnostic US study may obviate the need for CT, limiting repeated radiation to those with equivocal findings. MR enterography has become the imaging modality of choice for IBD in Europe, and advances in technique continue to drive adoption in the United States.84,90
In addition to becoming familiar with radiation risks associated with common CT studies, emergency physicians should be aware of the risks associated with repeated scanning. A recent study that estimated cumulative radiation exposure from CT of adults in a tertiary academic medical center found that one third of all patients underwent 5 or more CT during their lifetime and that 15% of all patients had estimated cumulative effective doses greater than 100 mSv.91 Another study found that those who obtained repeated CT imaging from a single ED increased their risk of developing cancer from their repeated exposure, and that the same study type represented most repeated imaging.86Furthermore, Kline et al34 determined that at least one third of ED patients who undergo CTPA to rule out PE will return for a second negative CTPA finding within 5 years.
As the public’s exposure to radiation from man-made sources has become a national topic of interest and concern, more and more patients will likely want to discuss possible radiation-induced cancer risks with their ED physicians before obtaining a CT. Moreover, as national governing bodies such as the FDA have classified ionizing radiation from medical imaging as a human carcinogen, patients are becoming more aware of the link between CT and cancer.92,93 The principles of medical ethics and patient autonomy dictate that ED physicians provide information regarding the risks, benefits, and alternatives of any given procedure to allow patients to make an informed decision.
Many physicians currently support the practice of obtaining informed consent for CT, including disclosure of minimal radiation-induced cancer risks.94 If physicians do speak with patients about such risks, they should do so in language that is understandable by the average patient. For instance, the magnitude of average effective doses for a particular CT could be expressed in terms of number of chest radiographs, transcontinental flights, or additional days of background exposure.93,95Furthermore, these risks should be expressed in light of the immediate benefits of obtaining a CT for any acute condition. It should also be stressed that any theoretic risk in increase of radiation-induced cancer from a single CT is actually a very small risk on top of the baseline cancer rate of 42% in the United States. For instance, since lifetime associated risk of fatal cancer from a single CT is estimated at around 0.1%, an abdominal CT effective dose is expected to increase the risk of developing cancer from 42% to 42.1%.23
Concerns that patients will refuse necessary examinations because of irrational fear of developing cancer have not been borne out in practice. In fact, patients may prefer to confirm their diagnosis with CT despite the radiation risks involved.96 Larson et al92 found that providing radiation-induced cancer risk information to parents of pediatric patients did not cause parents to refuse studies recommended by the referring physician. In another survey, nearly half of adult patients wanted to be informed about severe side effects with a 0.1% risk, whereas 13% of patients wished to be informed only when the risk reached 50% to 100%.97
LIMITATIONS
As discussed in the “Methods” section, this literature review does not cover the pediatric and pregnant female populations. These special patient populations are outside of the scope of this general primer. Our article does not discuss technical aspects of CT, which we believe are best left to radiologists, radiology technologists, and radiation physicists. In addition, most of the literature regarding radiation dose outside of the ED setting was deemed to be outside the scope for this article. Our literature review was based entirely on a MEDLINE search, as no additional articles specific to radiation dose in the ED setting were identified from additional databases such as Cochrane Review and Google Scholar.
CONCLUSION
While the ACR and similar organizations in Europe have developed appropriateness criteria for specific imaging studies, the emergency medicine community has yet to become intimately involved. Since emergency physicians are now responsible for a large and growing percentage of the overall CT volume, ED governing bodies must work closely with the radiology governing bodies to further develop clinical algorithms and guidelines to streamline the most effective use of CT in the ED. Moreover, many current guidelines are not geared toward the ED setting and, therefore, are of limited use to the emergency physician.
Recent studies have shown that ED physicians and patients alike are largely unaware of associated radiation-induced cancer risks from CT.93 Educational interventions may be effective for increasing such awareness. Stein et al98 demonstrated that practice patterns of physicians changed in response to an educational intervention including the emergency, radiology, and nuclear medicine departments at 1 institution, resulting in a reduction in radiation exposure to ED patients with suspected PE without compromising patient safety.
Recently, California became the first state to require the reporting of radiation exposure for every CT performed after reports of recent higher-than-normal radiation doses from CT stroke protocols at several southern California hospitals. If more states follow suit or if there is eventual national legislation mandating such measures, ED physicians will be responsible for using dose-level information in their clinical algorithms for repeated CT imaging. Innovative research opportunities currently exist for the use of electronic medical records and computer-assisted physician order entry systems for helping to track radiation dose from imaging in the ED setting. In all of these future patient safety endeavors, emergency physicians will play a central role in minimizing unnecessary radiation exposure and they should be versed in discussing the topic with their patients, radiologists, and consulting physicians.
Footnotes
Supervising Section Editor: Michael Burg, MD
Submission history: Submitted May 24, 2011; Revision received July 31, 2011; Accepted November 9, 2011
Reprints available through open access at http://escholarship.org/uc/uciem_westjem
DOI: 10.5811/westjem.2011.11.6804
Address for Correspondence: Jesse G.A. Jones, MD
USC Keck School of Medicine, Radiology Associates, 1520 San Pablo St, Lower Level, Los Angeles, CA 90033
E-mail: jessejon@usc.edu
Conflicts of Interest: By the WestJEM article submission agreement, all authors are required to disclose all affiliations, funding, sources, and financial or management relationships that could be perceived as potential sources of bias. The authors disclosed none.
REFERENCES
1. Brenner DJ, Doll R, Goodhead DT, et al. Cancer risks attributable to low doses of ionizing radiation: assessing what we really know. Proc Natl Acad Sci U S A. 2003;100:13761–13766. [PMC free article][PubMed]
2. Brenner DJ, Hall EJ. Computed tomography—an increasing source of radiation exposure. N Engl J Med. 2007;357:2277–2284. [PubMed]
3. Amis ES, Butler PF, Applegate KE, et al. American College of Radiology white paper on radiation dose in medicine. J Am Coll Radiol. 2007;4:272–284. [PubMed]
4. Wintermark M, Lev MH. FDA investigates the safety of brain perfusion CT. AJNR Am J Neuroradiol.2010;31:2–3. [PubMed]
5. Rumack CM. 2010 ACR presidential address: patient-focused radiology: taking charge of radiation dose. J Am Coll Radiol. 2010;7:837–844. [PubMed]
6. Bhargavan M. Trends in the utilization of medical procedures that use ionizing radiation. Health Phys. 2008;95:612–627. [PubMed]
7. Larson DB, Johnson LW, Schnell BM, et al. National trends in CT use in the emergency department: 1995–2007. Radiology. 2011;258:164–173. [PubMed]
8. Radiology ACo. Appropriateness criteria, 2004–2001. American College of Radiology Web site. Available at: http://www.acr.org/secondarymainmenucategories/quality_safety/app_criteria.aspx. Accessed April 28, 2011.
9. Bolus NE. Basic review of radiation biology and terminology. J Nucl Med Technol. 2001;29:67–73.test 76–77. [PubMed]
10. Dowd S, Tilson E. Practical Radiation Protection and Applied Radiobiology. 2nd ed. Philadelphia, PA: Saunders;; 1999.
11. ICRP. Radiation and your patient: a guide for medical practitioners. Ann ICRP. 2001;31:5–31.[PubMed]
12. NCRP. Evaluation of the Linear Nonthreshold Dose-Response Model for Ionizing Radiation.National Council on Radiation Protection and Measurements. Bethesda, MD: 2001.
13. Hall EJ, Brenner DJ. Cancer risks from diagnostic radiology. Br J Radiol. 2008;81:362–378.[PubMed]
14. Ventures IM. CT market summary report. 2006 IMVInfo Web site. Available at:http://www.imvinfo.com. Accessed November 13, 2010.
15. Frush DP, Applegate K. Computed tomography and radiation: understanding the issues. J Am Coll Radiol. 2004;1:113–119. [PubMed]
16. Pierce DA, Preston DL. Radiation-related cancer risks at low doses among atomic bomb survivors.Radiat Res. 2000;154:178–186. [PubMed]
17. Preston DL, Ron E, Tokuoka S, et al. Solid cancer incidence in atomic bomb survivors: 1958–1998.Radiat Res. 2007;168:1–64. [PubMed]
18. The National Academies Press Health risks from exposure to low levels of ionizing radiation: BEIR VII phase 2. Available at: http://www.nap.edu. Accessed November 8, 2010.
19. Berrington de González A, Mahesh M, Kim KP, et al. Projected cancer risks from computed tomographic scans performed in the United States in 2007. Arch Intern Med. 2009;169:2071–2077.[PubMed]
20. Ron E, Modan B, Preston D, et al. Thyroid neoplasia following low-dose radiation in childhood.Radiat Res. 1989;120:516–531. [PubMed]
21. Doody MM, Lonstein JE, Stovall M, et al. Breast cancer mortality after diagnostic radiography: findings from the U.S. Scoliosis Cohort Study. Spine (Phila Pa 1976) 2000;25:2052–2063. [PubMed]
22. The 2007 recommendations of the International Commission on Radiological Protection. ICRP publication 103. Ann ICRP. 2007;37((2–4)):1–332.
23. Smith-Bindman R, Lipson J, Marcus R, et al. Radiation dose associated with common computed tomography examinations and the associated lifetime attributable risk of cancer. Arch Intern Med.2009;169:2078–2086. [PubMed]
24. Brenner DJ, Elliston CD. Estimated radiation risks potentially associated with full-body CT screening. Radiology. 2004;232:735–738. [PubMed]
25. Mettler FA, Huda W, Yoshizumi TT, et al. Effective doses in radiology and diagnostic nuclear medicine: a catalog. Radiology. 2008;248:254–263. [PubMed]
26. Shrimpton PC, Hillier MC, Lewis MA, et al. National survey of doses from CT in the UK: 2003. Br J Radiol. 2006;79:968–980. [PubMed]
27. Diederich S, Lenzen H. Radiation exposure associated with imaging of the chest: comparison of different radiographic and computed tomography techniques. Cancer. 2000;89((11 suppl)):2457–2460.[PubMed]
28. Huda W, Vance A. Patient radiation doses from adult and pediatric CT. AJR Am J Roentgenol.2007;188:540–546. [PubMed]
29. Wells PS, Anderson DR, Rodger M, et al. Excluding pulmonary embolism at the bedside without diagnostic imaging: management of patients with suspected pulmonary embolism presenting to the emergency department by using a simple clinical model and d-dimer. Ann Intern Med. 2001;135(2):98–107. [PubMed]
30. van Belle A, Büller HR, Huisman MV, et al. Effectiveness of managing suspected pulmonary embolism using an algorithm combining clinical probability, D-dimer testing, and computed tomography. JAMA. 2006;295:172–179. [PubMed]
31. Le Gal G, Righini M, Roy PM, et al. Prediction of pulmonary embolism in the emergency department: the revised Geneva score. Ann Intern Med. 2006;144:165–171. [PubMed]
32. Wells PS, Owen C, Doucette S, et al. Does this patient have deep vein thrombosis? JAMA.2006;295:199–207. [PubMed]
33. Schoepf UJ, Costello P. CT angiography for diagnosis of pulmonary embolism: state of the art.Radiology. 2004;230:329–337. [PubMed]
34. Kline JA, Courtney DM, Kabrhel C, et al. Prospective multicenter evaluation of the pulmonary embolism rule-out criteria. J Thromb Haemost. 2008;6:772–780. [PubMed]
35. Drescher FS, Chandrika S, Weir ID, et al. Effectiveness and acceptability of a computerized decision support system using modified Wells criteria for evaluation of suspected pulmonary embolism. Ann Emerg Med. 2011;57:613–621. [PubMed]
36. Fesmire FM, Brown MD, Espinosa JA, et al. Critical issues in the evaluation and management of adult patients presenting to the emergency department with suspected pulmonary embolism. Ann Emerg Med. 2011;57:628–652. e75. [PubMed]
37. Buenger RE. Five thousand acute care/emergency department chest radiographs: comparison of requisitions with radiographic findings. J Emerg Med. 1988;6:197–202. [PubMed]
38. Greenspan RH, Ravin CE, Polansky SM, et al. Accuracy of the chest radiograph in diagnosis of pulmonary embolism. Invest Radiol. 1982;17:539–543. [PubMed]
39. Stein PD, Kayali F, Olson RE. Trends in the use of diagnostic imaging in patients hospitalized with acute pulmonary embolism. Am J Cardiol. 2004;93:1316–1317. [PubMed]
40. Stein PD, Fowler SE, Goodman LR, et al. Multidetector computed tomography for acute pulmonary embolism. N Engl J Med. 2006;354:2317–2327. [PubMed]
41. Schoepf UJ, Holzknecht N, Helmberger TK, et al. Subsegmental pulmonary emboli: improved detection with thin-collimation multi-detector row spiral CT. Radiology. 2002;222:483–490. [PubMed]
42. Einstein AJ, Henzlova MJ, Rajagopalan S. Estimating risk of cancer associated with radiation exposure from 64-slice computed tomography coronary angiography. JAMA. 2007;298:317–323.[PubMed]
43. Parker MS, Hui FK, Camacho MA, et al. Female breast radiation exposure during CT pulmonary angiography. AJR Am J Roentgenol. 2005;185:1228–1233. [PubMed]
44. Task Group on Control of Radiation Dose in Computed Tomography. Managing patient dose in computed tomography. A report of the International Commission on Radiological Protection. Ann ICRP. 2000;30:7–45.
45. Freeman LM, Stein EG, Sprayregen S, et al. The current and continuing important role of ventilation-perfusion scintigraphy in evaluating patients with suspected pulmonary embolism. Semin Nucl Med. 2008;38:432–440. [PubMed]
46. Hurwitz LM, Yoshizumi T, Reiman RE, et al. Radiation dose to the fetus from body MDCT during early gestation. AJR Am J Roentgenol. 2006;186:871–876. [PubMed]
47. Teichman JM, Long RD, Hulbert JC. Long-term renal fate and prognosis after staghorn calculus management. J Urol. 1995;153:1403–1407. [PubMed]
48. Ha M, MacDonald RD. Impact of CT scan in patients with first episode of suspected nephrolithiasis.J Emerg Med. 2004;27:225–231. [PubMed]
49. Smith RC, Verga M, McCarthy S, et al. Diagnosis of acute flank pain: value of unenhanced helical CT. AJR Am J Roentgenol. 1996;166:97–101. [PubMed]
50. Heneghan JP, McGuire KA, Leder RA, et al. Helical CT for nephrolithiasis and ureterolithiasis: comparison of conventional and reduced radiation-dose techniques. Radiology. 2003;229:575–580.[PubMed]
51. Poletti PA, Platon A, Rutschmann OT, et al. Low-dose versus standard-dose CT protocol in patients with clinically suspected renal colic. AJR Am J Roentgenol. 2007;188:927–933. [PubMed]
52. Tack D, Sourtzis S, Delpierre I, et al. Low-dose unenhanced multidetector CT of patients with suspected renal colic. AJR Am J Roentgenol. 2003;180:305–311. [PubMed]
53. Liu W, Esler SJ, Kenny BJ, et al. Low-dose nonenhanced helical CT of renal colic: assessment of ureteric stone detection and measurement of effective dose equivalent. Radiology. 2000;215:51–54.[PubMed]
54. Hamm M, Knopfle E, Wartenberg S, et al. Low dose unenhanced helical computerized tomography for the evaluation of acute flank pain. J Urol. 2002;167:1687–1691. [PubMed]
55. Kim BS, Hwang IK, Choi YW, et al. Low-dose and standard-dose unenhanced helical computed tomography for the assessment of acute renal colic: prospective comparative study. Acta Radiol.2005;46:756–763. [PubMed]
56. Hyams ES, Shah O. Evaluation and follow-up of patients with urinary lithiasis: minimizing radiation exposure. Curr Urol Rep. 2010;11:80–86. [PubMed]
57. Sheafor DH, Hertzberg BS, Freed KS, et al. Nonenhanced helical CT and US in the emergency evaluation of patients with renal colic: prospective comparison. Radiology. 2000;217:792–797.[PubMed]
58. Patlas M, Farkas A, Fisher D, et al. Ultrasound vs CT for the detection of ureteric stones in patients with renal colic. Br J Radiol. 2001;74:901–904. [PubMed]
59. Ripollés T, Errando J, Agramunt M, et al. Ureteral colic: US versus CT. Abdom Imaging.2004;29:263–266. [PubMed]
60. Catalano O, Nunziata A, Altei F, et al. Suspected ureteral colic: primary helical CT versus selective helical CT after unenhanced radiography and sonography. AJR Am J Roentgenol. 2002;178:379–387.[PubMed]
61. Svedström E, Alanen A, Nurmi M. Radiologic diagnosis of renal colic: the role of plain films, excretory urography and sonography. Eur J Radiol. 1990;11:180–183. [PubMed]
62. Ripollés T, Agramunt M, Errando J, et al. Suspected ureteral colic: plain film and sonography vs unenhanced helical CT: a prospective study in 66 patients. Eur Radiol. 2004;14:129–136. [PubMed]
63. Wieseler KM, Bhargava P, Kanal KM, et al. Imaging in pregnant patients: examination appropriateness. Radiographics. 2010;30:1215–1222. discussion 1230–1233. [PubMed]
64. Baumgarten D, Francis I, Casalino D, et al. Acute onset flank pain—suspicion of stone disease.American College of Radiology Web site. Available at: http://acsearch.acr.org/TopicList.aspx?topic_all=&topic_any=%27%22renal+failure*%22%27&connector=+And+&cid=0http://acsearch.acr.org/TopicList.aspx?topic_all=&topic_any=%27%22renal+failure*%22%27&connector=+And+&cid=0. Accessed February 12, 2011.
65. John BS, Patel U, Anson K. What radiation exposure can a patient expect during a single stone episode? J Endourol. 2008;22:419–422. [PubMed]
66. Katz SI, Saluja S, Brink JA, et al. Radiation dose associated with unenhanced CT for suspected renal colic: impact of repetitive studies. AJR Am J Roentgenol. 2006;186:1120–1124. [PubMed]
67. Broder J, Bowen J, Lohr J, et al. Cumulative CT exposures in emergency department patients evaluated for suspected renal colic. J Emerg Med. 2007;33:161–168. [PubMed]
68. Goldstone A, Bushnell A. Does diagnosis change as a result of repeat renal colic computed tomography scan in patients with a history of kidney stones? Am J Emerg Med. 2010;28:291–295.[PubMed]
69. Modahl L, Digumarthy SR, Rhea JT, et al. Emergency department abdominal computed tomography for nontraumatic abdominal pain: optimizing utilization. J Am Coll Radiol. 2006;3:860–866. [PubMed]
70. Scheinfeld MH, Mahadevia S, Stein EG, et al. Can lab data be used to reduce abdominal computed tomography (CT) usage in young adults presenting to the emergency department with nontraumatic abdominal pain? Emerg Radiol. 2010;17:353–360. [PubMed]
71. Abdullah BA, Gupta SK, Croffie JM, et al. The role of esophagogastroduodenoscopy in the initial evaluation of childhood inflammatory bowel disease: a 7-year study. J Pediatr Gastroenterol Nutr.2002;35:636–640. [PubMed]
72. Hemminki K, Li X, Sundquist J, et al. Cancer risks in Crohn disease patients. Ann Oncol.2009;20:574–580. [PubMed]
73. Palmer L, Herfarth H, Porter CQ, et al. Diagnostic ionizing radiation exposure in a population-based sample of children with inflammatory bowel diseases. Am J Gastroenterol. 2009;104:2816–2823.[PMC free article] [PubMed]
74. Desmond AN, O’Regan K, Curran C, et al. Crohn’s disease: factors associated with exposure to high levels of diagnostic radiation. Gut. 2008;57:1524–1529. [PubMed]
75. Huprich JE, Rosen MP, Fidler JL, et al. ACR appropriateness criteria on Crohn’s disease. J Am Coll Radiol. 2010;7:94–102. [PubMed]
76. Mann EH. Inflammatory bowel disease: imaging of the pediatric patient. Semin Roentgenol.2008;43:29–38. [PubMed]
77. Miao YM, Koh DM, Amin Z, et al. Ultrasound and magnetic resonance imaging assessmentof active bowel segments in Crohn’s disease. Clin Radiol. 2002;57:913–918. [PubMed]
78. Spalinger J, Patriquin H, Miron MC, et al. Doppler US in patients with crohn disease: vessel density in the diseased bowel reflects disease activity. Radiology. 2000;217:787–791. [PubMed]
79. Bremner AR, Griffiths M, Argent JD, et al. Sonographic evaluation of inflammatory bowel disease: a prospective, blinded, comparative study. Pediatr Radiol. 2006;36:947–953. [PubMed]
80. Maconi G, Sampietro GM, Parente F, et al. Contrast radiology, computed tomography and ultrasonography in detecting internal fistulas and intra-abdominal abscesses in Crohn’s disease: a prospective comparative study. Am J Gastroenterol. 2003;98:1545–1555. [PubMed]
81. Saibeni S, Rondonotti E, Iozzelli A, et al. Imaging of the small bowel in Crohn’s disease: a review of old and new techniques. World J Gastroenterol. 2007;13:3279–3287. [PubMed]
82. Sempere GA, Martinez Sanjuan V, Medina Chulia E, et al. MRI evaluation of inflammatory activity in Crohn’s disease. AJR Am J Roentgenol. 2005;184:1829–1835. [PubMed]
83. Koh DM, Miao Y, Chinn RJ, et al. MR imaging evaluation of the activity of Crohn’s disease. AJR Am J Roentgenol. 2001;177:1325–1332. [PubMed]
84. Schreyer AG, Seitz J, Feuerbach S, et al. Modern imaging using computer tomography and magnetic resonance imaging for inflammatory bowel disease (IBD) AU1. Inflamm Bowel Dis.2004;10:45–54. [PubMed]
85. Rankey D, Leach JL, Leach SD. Emergency MRI utilization trends at a tertiary care academic medical center: baseline data. Acad Radiol. 2008;15:438–443. [PubMed]
86. Griffey RT, Sodickson A. Cumulative radiation exposure and cancer risk estimates in emergency department patients undergoing repeat or multiple CT. AJR Am J Roentgenol. 2009;192:887–892.[PubMed]
87. Levin DC, Rao VM, Parker L, et al. Recent shifts in place of service for noninvasive diagnostic imaging: have hospitals missed an opportunity? J Am Coll Radiol. 2009;6:96–99. [PubMed]
88. Sharp HT. The acute abdomen during pregnancy. Clin Obstet Gynecol. 2002;45:405–413.[PubMed]
89. Pedrosa I, Levine D, Eyvazzadeh AD, et al. MR imaging evaluation of acute appendicitis in pregnancy. Radiology. 2006;238:891–899. [PubMed]
90. Schwartz DA, Wiersema MJ, Dudiak KM, et al. A comparison of endoscopic ultrasound, magnetic resonance imaging, and exam under anesthesia for evaluation of Crohn’s perianal fistulas.Gastroenterology. 2001;121:1064–1072. [PubMed]
91. Sodickson A, Baeyens PF, Andriole KP, et al. Recurrent CT, cumulative radiation exposure, and associated radiation-induced cancer risks from CT of adults. Radiology. 2009;251:175–184. [PubMed]
92. Larson DB, Rader SB, Forman HP, et al. Informing parents about CT radiation exposure in children: it’s OK to tell them. AJR Am J Roentgenol. 2007;189:271–275. [PubMed]
93. Lee CI, Haims AH, Monico EP, et al. Diagnostic CT scans: assessment of patient, physician, and radiologist awareness of radiation dose and possible risks. Radiology. 2004;231:393–398. [PubMed]
94. Karsli T, Kalra MK, Self JL, et al. What physicians think about the need for informed consent for communicating the risk of cancer from low-dose radiation. Pediatr Radiol. 2009;39:917–925. [PubMed]
95. Baerlocher MO, Detsky AS. Discussing radiation risks associated with CT scans with patients. JAMA.2010;304:2170–2171. [PubMed]
96. Takakuwa KM, Estepa AT, Shofer FS. Knowledge and attitudes of emergency department patients regarding radiation risk of CT: effects of age, sex, race, education, insurance, body mass index, pain, and seriousness of illness. AJR Am J Roentgenol. 2010;195:1151–1158. [PubMed]
97. Barnett GC, Charman SC, Sizer B, et al. Information given to patients about adverse effects of radiotherapy: a survey of patients’ views. Clin Oncol (R Coll Radiol) 2004;16:479–484. [PubMed]
98. Stein EG, Haramati LB, Chamarthy M, et al. Success of a safe and simple algorithm to reduce use of CT pulmonary angiography in the emergency department. AJR Am J Roentgenol. 2010;194:392–397.[PubMed]