Author | Affiliation |
---|---|
Steven C. Gabaeff, MD | Emergency Medicine and Clinical Forensic Medicine, Sacramento, CA |
ABSTRACT
Child abuse experts use diagnostic findings of subdural hematoma and retinal hemorrhages as near-pathognomonic findings to diagnose shaken baby syndrome. This article reviews the origin of this link and casts serious doubt on the specificity of the pathophysiologic connection. The forces required to cause brain injury were derived from an experiment of high velocity impacts on monkeys, that generated forces far above those which might occur with a shaking mechanism. These forces, if present, would invariably cause neck trauma, which is conspicuously absent in most babies allegedly injured by shaking. Subdural hematoma may also be the result of common birth trauma, complicated by prenatal vitamin D deficiency, which also contributes to the appearance of long bone fractures commonly associated with child abuse. Retinal hemorrhage is a non-specific finding that occurs with many causes of increased intracranial pressure, including infection and hypoxic brain injury. The evidence challenging these connections should prompt emergency physicians and others who care for children to consider a broad differential diagnosis before settling on occult shaking as the de-facto cause. While childhood non-accidental trauma is certainly a serious problem, the wide exposure of this information may have the potential to exonerate some innocent care-givers who have been convicted, or may be accused, of child abuse.
INTRODUCTION
For many years the relationship between retinal hemorrhage (RH) and the shaken baby syndrome (SBS) has been accepted as fact and widely used to diagnose children as victims of child abuse. (Please see Table 1 for a list of abbreviations used in this manuscript)
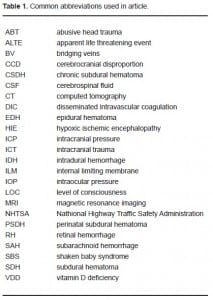
The scientific literature, both old and new, has cultivated the belief that RH is uniquely linked and virtually pathognomonic of shaking and nonaccidental trauma. The child abuse community, including most pediatricians and ophthalmologists, assume that the presence of RH proves that a child was shaken abusively. Donohoe1 graded the quality of the child abuse literature from 1966 to 1998 and found significant weaknesses, concluding that there was inadequate scientific evidence to come to “a firm conclusion on most matters pertaining to SBS.” He called appropriately for controlled, prospective trials into SBS and opined: “Without published and replicated studies of that type the commonly held opinion that the finding of subdural hematoma and RH in an infant was strong evidence of SBS was unsustainable, at least from the medical literature.”
The established assumption has been that shaking directly and independently damages capillaries in the eye and tears bridging veins in the brain, causing hemorrhage.2 In the absence of overt brain damage, RH and subdural hematoma (SDH) have emerged as sufficient criteria necessary to diagnose SBS. Furthermore, either one at times has been used independently to make the diagnosis and initiate criminal proceedings. However, both SDH and RH can result from a number of other traumatic or medical conditions. This paper will challenge the rationale behind the exclusive link between RH, SDH and child abuse, and whether vessels in the cranial vault and eye are damaged by a shaking mechanism.
The History of Shaken Baby Syndrome
SBS was first theorized by Guthkelch and later Caffey who published in the 1970s. They proposed the concept of “Whiplash Shaken Infant Syndrome” in two published articles,2,3 which extrapolated from an experiment on monkeys by A.K. Ommaya4, a neurosurgeon. Ommaya4, in 1968, looked at the substantial head and neck injuries in rhesus monkeys subjected to whiplash in simulated 40 mph rear end motor vehicle “collisions” using a mechanical piston.
Caffey2 and Guthkelch3, without further independent investigation, theorized that human shaking could cause intracranial injury similar to Ommaya’s monkeys. To explain the RHs that were often present with SDH, Caffey2 advanced that RH would result from human shaking by damaging retinal capillaries.
This theory explained the findings of RH and SDH in patients with, in their view, inadequate mechanisms to account for “severe head injury.” They therefore proposed another explanation: that clandestine shaking could produce the combination of SDH and RH. Their theory did not consider the significant literature (discussed below) that had established that intracranial hemorrhage and increased intracranial pressures (ICP), without impact or shaking, are well-documented causes of RH. The new construct was published and subsequently advanced in large-scale conferences aimed at law enforcement, social services and physicians. Those who accepted this theory came to believe that violent human shaking and high speed impacts would produce similar physical findings.
Ommaya’s Study: Physics of Shaking in the Brain and Eye
On this basis it is instructive to examine Ommaya’s study, which became the biomechanical basis of “shaken whiplash,” and later “shaken baby” syndrome. Using rhesus monkeys, he attempted to quantify the “rotational acceleration” (i.e. whiplash) that was necessary to cause loss of consciousness. Whiplash is defined as a rotational force, with the head rotating around a point in the lower cervical spine in an arc.4 Rotational force is calculated from the speed of rotation measured in degrees per unit of time (rotational velocity), and the distance from the point of rotation (lower neck) to the center of gravity of the rotating object (the head). In Ommaya’s study, after a single, forceful impact, he documented loss of consciousness, recovery and autopsy findings (Figure 1).
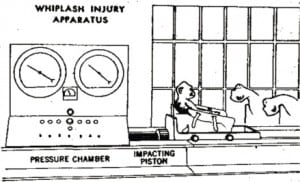
Ommaya’s monkeys were strapped to a chair on a sled with unrestricted head movements. Impacts to the back of the chair produced significant accelerations, which can be measured in Gs. One G is the acceleration (change of velocity) due to gravity of an object falling in our atmosphere when air resistance is negligible. It is a constant at 32.2 ft/sec2, a number that connotes an exponential change in speed. In physics this is “acceleration.” Linear (straight line) acceleration can be measured in Gs or in ft/sec2, and rotational accelerations (movement around a center point in an arc) can also be measured in Gs or in radians/sec2, where a radian is equal to about 57 degrees of arc (about 1/6thof a full circle). Acceleration is the rate of change in velocity of any object under a variety of circumstances, including falls, impacts and shaking. When velocity is increasing, we measure “acceleration,” and when decreasing, “negative acceleration,” which is often referred to by the more common term “deceleration.” The time it takes to change from one velocity to another is the deceleration time.
Ommaya subjected the monkeys to a very forceful whiplash: 40,000 radians/sec2 of rotational acceleration to the head. In these monkeys, with the distance from the base of the neck to the center of gravity of the head estimated at 6 inches, it was the equivalent of about 600 Gs or 600 times the acceleration due to gravity. Based on National Highway Traffic Safety Administration (NHTSA) data, known injury thresholds to the brain are in the 80–100 Gs range.5 Above 100 Gs, injury to the brain can be expected. Below 50 Gs no injury occurs.6
After impact, Ommaya looked at autopsy findings of the 19 subjects that lost consciousness with the whiplash. Fifteen (79%) had significant gross SDH, and eight of the 19 (42%) had gross evidence of severe neck injury with visible hemorrhage to the brainstem surface. In addition, Ommaya7,8separately studied soft tissue and histologic damage, and neurologic dysfunction, which were widespread and considerable in the brain stem.
With current technology, these neck findings following whiplash injury would be evident as soft tissue swelling from hematoma or edema on magnetic resonance image (MRI) and computed tomography (CT) of the neck. The consistent association of SDH and RH with neck damage that Ommaya found is important because shaking forces reach the head via the neck, the weak link between torso and head movement. In recent years, as MRI has become routine in child abuse evaluation, neck pathology has not been found in virtually all cases of alleged SBS.8,9 In Bandak’s10study of projected neck damage from abusive shaking he stated, “We have determined that an infant head subjected to the levels of rotational velocity and acceleration called for in the SBS literature, would experience forces on the infant neck far exceeding the limits for structural failure of the cervical spine. Furthermore, shaking cervical spine injury can occur at much lower levels of head velocity and acceleration than those reported for the SBS.”
Technologically advanced biomechanical testing with sophisticated instrumented mannequins have failed to demonstrate that abusive shaking of an infant can generate comparable G forces to Ommaya’s impacts, or from common household falls over three feet, as calculated below. Testing has shown humans can generate only about 10–15 Gs of acceleration of the brain with the most forceful shaking, a fraction of Ommaya’s 600 Gs, and well below the NHTSA’s acknowledged threshold for injury.11,12 The strength of the infant neck increases in a linear manner, with the newborn most vulnerable to abusive shaking. Bandak10 showed that neck failure, across a spectrum of ages for infants, will occur before brain injury. Barnes13 more recently used his MRI experience at Stanford to further document the lack of neck findings with alleged shaking and reaffirmed the expectation of neck pathology with a substantial shaking mechanism, including soft tissue injury, ligament damage, fractures and in the extreme, decapitation.14,15
Children may be shaken to minor degrees in the course of child care and during well-meaning attempts at resuscitation. Caregivers use various bouncing behaviors to console and entertain children (swinging, rocking, lifting, throwing), and minor resuscitative “shakes” are taught in cardiopulmonary resuscitation classes as “checks for responsiveness.” These reports of shaking, in the author’s experience, are often regarded by police and child abuse specialists as “confessions of shaking.”16 However, they involve simple translational (side to side) motion and using biomechanical analysis, have been shown to produce low single-digit G accelerations.17 The level of force is equivalent to that during child’s play and normal handling. In a 2001 report by the National Association of Medical Examiners Ad Hoc Committee on SBS, these forces were acknowledged to be “quite benign”, yet these admissions are used by prosecutors and child abuse professionals as confessions of abusive shaking, even when the force generated is known to be minimal.17
Thus, based on analysis of the force required to cause intracranial injury and the impact of shaking on the neck, without some findings of neck injury on imaging, intracranial pathology resulting from human shaking of a previously healthy child should be seriously called into question.
Other studies looking at accelerations and the alleged relationship between acceleration and RH have similarly shown that high accelerations do not cause RH. Funk18 looked at 26,000 instrumented football helmet collisions measured during games, with about 2000 collisions generating >85 Gs (or >6000rad/sec2) of force each. While serious injury occurs in football, this study measured force with routine contact. In this study, the four most severe of the 26,000 collisions resulted in mild traumatic brain injury (concussion). No other injuries, including SDH or RH, occurred to any other players. In this study the threshold for a 10% chance of injury to occur was established at 165 Gs. Animal experimentation generating extreme accelerations to the eye without impact at around 1000 Gs has also failed to produce any RH.19,20 These accelerations are 70–100 times greater than those that can be generated by humans through a shaking mechanism.
Case reports of witnessed or videotaped shaking of a previously healthy child with demonstrated RH or SDH upon immediate evaluation are conspicuously absent from a thorough search of the forensic and medical literature. Conversely, shaking episodes have been recorded, but have not been associated with SBS injury markers (see link with “References” below to download and view “shaking without injury videos).
If human shaking is not responsible for cases of RH, then what are the mechanisms associated with it? It seems clear that RH does not occur in pure high G situations. What will be explored first is the long-known relationship of increased intracranial pressure (ICP) to RH. Other possible mimics to alleged abuse and their particular findings will be discussed.
Impact trauma may be the common etiology of increased ICP, SDH and other neuropathologic findings. This connection requires comparison of forces in impact vs. shaking to determine if the data further undermines the link with SBS.
Calculation of Force with Impact and Clinical Considerations
The intensity of force related to shaking or impact is related to (1) change in velocity, (2) the deceleration time and (3) the mass of the object in motion. Force declines rapidly as deceleration time increases by even fractions of a second. A pole vaulter who drops 20 feet and lands in a deep foam pit with a one-second (1000 milliseconds) deceleration time, which is long in physics terms, is not injured. Conversely, extremely rapid changes in velocity on the order of 7.5 milliseconds, from either rapid accelerations like Ommaya’s rear end impact experiments or rapid decelerations like a fall to a hard surface, both produce much higher G forces. For example, when a four-pound head hits a concrete floor from three feet high, it would be moving at 13.9 feet/second and would stop in about 7.5 milliseconds (a standard time for a moving skull hitting a hard surface to reach zero velocity). This almost-instantaneous change produces a peak deceleration of 130 Gs. To calculate force at impact the mass of the object must be considered and assuming a four-pound head (about average for a 15–20 pound baby), the peak force at impact is about 265 pounds. To underscore the nonlinear increase of Gs at impact with small changes in velocity, when the same four-pound head hits concrete from just 18 inches higher (4 feet 6 inches), it is traveling at 17 feet/second and generates 170 Gs and 360 pounds of force*. If there is rotation at the time of impact (rotating and falling), velocity attributed to gravity and rotational velocity is cumulative and “resultant” forces increase as well. The most conservative force calculations assume a single part like the head, dropping straight down with no rotation.
While biomechanical analysis supports the mechanistic link between falls and high impact, clinically, short falls have been documented to cause serious injury. Greenes et al.21 in 1997 reported that among children with falls of less than three feet brought to an ED approximately 18% had severe intracranial trauma (ICT) or skull fracture. Another of Greenes’22 studies in 1998 found 19% of children with significant head injury, primarily skull fractures, were “occult,” without neurologic symptoms or evidence of external trauma.
On this basis, the consideration of intentional impact must be carefully evaluated to diagnose abuse, as it is clear that short falls in household situations are sufficient to cause not only ICT, but even death.23
Bridging Veins and the Relationship to Subdural Hematoma in Healthy Children
Integral to the SBS hypothesis has been the concept that movement of the brain during shaking shears off the large veins between the brain and the dura, causing SDH. These large veins, the bridging veins (BV), are substantial and move blood from the brain to the dural sinuses. (Figures 2aand and2b)2b) There are about 10 to 20 BVs between the brain and the dura.24
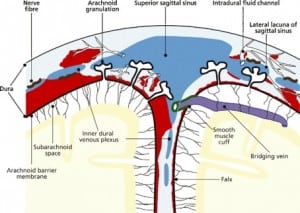
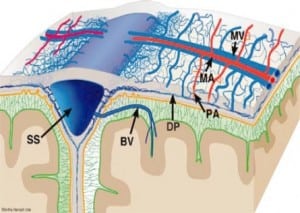
Autopsy photos show that BVs are large, appear to stretch substantially and even with severe cerebrocranial disproportion (CCD, extra space around the brain), restriction of movement remains substantial25 (Figure 3).
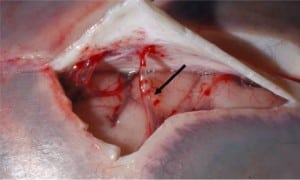
Furthermore, if torn BVs were the cause of SDH formation there would be large collections of blood in SBS cases at autopsy or on CT in all SBS cases. This picture is virtually never seen in alleged SBS.26Instead we see smaller collections of blood in the subdural space from only intradural hemorrhage (IDH) from “thin film” to about 1 cm thick. Furthermore, larger bleeds, when found, are relatively contained due to lower capillary pressures, as compared to BVs with higher pressures that increase the mass of extravasated blood. The result would be massive SDH as demonstrated in Figure 4.
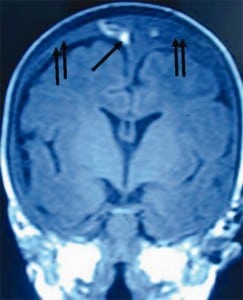
BVs can be torn in severe head trauma when depressed skull fractures intrude into the brain space or with extreme CCD. The CCD occurs with severe atrophy in the elderly or in infants with previous birth-related SDH. In either case, a large subarachnoid space between the brain and skull can stretch BV to their tensile limit with even minor movement. If the limits are exceeded and the veins fail, the end result is massive SDH. Therefore, tearing of BVs is an unlikely a cause of SDH in previously healthy infant, but may play some role in the rebleed of an infant with severe CCD from previous SDH, chronic subdural hematoma (CSDH) and significant hygroma.
These situations, however, are distinctly different from the neurologic state of a healthy child allegedly being shaken or the victim of impact trauma. Calculations of flow capacity through a single BV has been studied in elderly adults with micro-ultrasound Doppler techniques, showing that each vein carries on average about 5 cc/min of blood.27 Thus, when BVs tear, the result is substantial hemorrhage in a very short time with a grave consequences (Figure 4).
The Pathophysiology of Subdural Hematoma
If tearing of BVs in healthy children via shaking is not plausible and intrusive brain injury has not occurred, then what different mechanisms and etiologies can produce SDH in healthy children?
We know that impact trauma can cause SDH; hence, a closer examination of the intricate layers covering the brain may reveal that SDH can occur without the tearing of BVs. It is now clear that that SDH begins as IDH and is caused by physical or physiologic damage to the dural capillary plexus.28IDH can occur in response to a variety of primary insults. However, if a child has a preexisiting SDH of any etiology, and chronic SDH has developed, shaking or even normal handling can result in spontaneous rebleeding of the previous SDH (subacute or chronic SDH). Vinchon et al29 in 2010 found 10% of all SDH cases over a three year period at his institution (16 children total) had spontaneous rebleeds without evidence of abuse. Again the distinction between the previously healthy child and the previously damaged child must be made.
These traumatic etiologies in any child, however, are not the only etiology of dural bleeding. Cohen and Scheimberg30 in 2008, demonstrated that “SDH and cerebral hypoxia are common associations of IDH and that SDH (often seen as a thin film of hemorrhage) almost always occur in association with diffuse falcine IDH. Diffuse IDH with SDH are more frequently associated with severe or moderate hypoxic ischemic encephalopathy (HIE), while mild or early HIE is more common with focal IDH without SDH.” Their findings in cases clearly not involving abuse of any type, often occurred in the context of documented increased ICP, acidosis, coagulopathies and/or sepsis, among other medical neuropathologies. Destruction of brain tissue in meningitis and encephalitis and disseminated intravascular coagulation (DIC) are among other things that commonly result in SDH, subarachnoid hemorrhage (SAH), intraparenchymal hemorrhage and intraventricular hemorrhage.31
In all cases of SDH in a previously healthy child, IDH occurs first since the capillaries of the dural venous plexus are the source of blood in SDH (Figure 2b). When copious, the hemorrhage overflows the intradural space to fill the “potential” subdural space between the easily cleavable and porous dural border cell layer and the arachnoid. The SDH forms in this newly created “subdural” space (Figures 5a, ,5b and 5c).
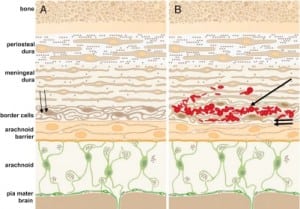
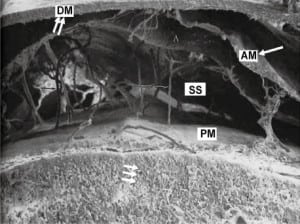
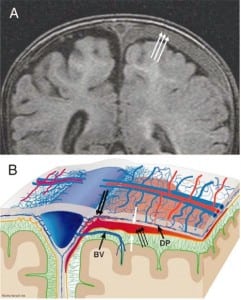
Pediatric child abuse specialists have acknowledged that a large number of children fall each day and have posited that only a small number of short falls, perhaps “one in 1 million,” produce serious or at times fatal injuries.32 With a large pediatric population, we can assume that serious falls will occur every day. As we know, most parents will seek medical attention for their children after only the more frightening accidental fall. We also know that about one in six of these frightening falls result in serious injury.21,22 On this basis, it is illogical to reflexively assume a different, sinister act has occurred in patients who are found to have SDH after an accidental fall. Rather, we should recognize that a very small subset of all accidental falls can and do result in serious brain injury. With a large denominator of accidental falls, the serious brain injuries can and do result from innocent, accidental mechanisms, and each of these cases most likely prompts a medical encounter.
The nature of the serious fall, as compared to the large number of non-serious falls, is that the baby must land just so, with a vulnerable part (i.e. skull) taking the brunt of the impact at a sufficient speed, against a sufficiently hard surface to generate the requisite forces. Most importantly, with head impacts, every time these criteria are reached, serious injury results: SDH, skull fractures, epidural hematoma (EDH), RH, cerebral edema and other injuries are expected. Yet Caffey23 and his cohorts presumed from the inception of the SBS theory that short falls could not produce adequate force to cause serious injury. This was incorrect.23 Now with both new and old research,33 some child abuse specialists will for the first time admit that short falls can cause serious injury, including SDH, and have recently acknowledged that RH and other retinal damage can occur with short falls as well.34–37
Relation of Increased Intracranial Pressure to Retinal Hemorrhage
There is a long and well-documented relationship of RH to increased ICP unrelated to abuse. The non-abuse causes of increased ICP leading to RH can be due to infection, hypoxicischemic encephalopathy (HIE), post-event cerebral edema from any traumatic or metabolic calamity and in some cases an idiopathic etiology.
The first of many studies to show that RH is associated with increased ICP of any etiology was published in 1900 by Dr. Albert Terson38, a French ophthalmologist. Terson’s syndrome, as it came to be known, showed that increased ICP after hemorrhagic stroke produced RHs. Medele39 in 1988 revalidated Terson’s original study. More recent studies have done the same.40
Walsh41 in 1951 demonstrated the relationship of increase ICP with optic nerve sheath hemorrhage and RH. The optic nerve sheath is the dura surrounding the optic nerve, and the mechanism that produces optic nerve sheath hemorrhage, IDH and RH, is the same. Muller41 in 1974 confirmed that increased ICP caused optic nerve sheath hemorrhage. Aoki43 in 1986 found that 100% of children, not shaken, with SDH from impact trauma, had RH. Lashutka44 in 2004 plotted a 1:1 correlation between increased ICP and increased intraocular pressure. Tayal46 in 2007 found that optic nerve diameter, measured by ultrasound, nearly doubled in size when increased ICP was present after impact trauma that resulted in increased ICP. Increasing intraocular pressure (IOP) decreases flow through the eye by increasing intracapillary pressure, decreasing both ingress and egress of blood from the eye, contributing to the metabolic compromises, capillary wall damage and leakage of blood, and RH.
Other etiologies of RH include transient increases in ICP with extreme coughing and valsalva, which can occur with choking episodes.47–48 High altitude cerebral edema, another form of protracted hypoxemia, also produces RH.49 RH from the compressive forces of labor alone raising ICP in the head, occurs in 45% of all births.50
It is clear that RH occurs from many causes and that shaking, if capable of causing RH, is quite nonspecific.
Pathophysiology of Retinal Hemorrhages, Schisis Cavities and Vitreous Hemorrhage
A closer examination of RH itself supports the relationship of RH to brain pathology with increased ICP. RH could be regarded as the first finding in a continuum of ocular hemorrhages associated with brain pathology and increased ICP, not specifically from shaking, or even a traumatic mechanism.
As ICP increases, IOP also increases.44,46 As IOP increases, intraocular capillary perfusion pressure decreases and oxygen delivery to the retina falls. This is similar to the well-accepted mechanism that a decrease in cerebral perfusion pressure occurs in the cranium as ICP rises. In the brain, this leads to metabolic catastrophe, HIE and brain death. Similarly, when capillary blood flow to the eye decreases, a relative or absolute hypoxemia occurs in the retinal capillary cell wall, damaging the capillary wall that then leaks, forming RH.51 This is the same mechanism that creates IDH and SDH during harmful hypoxic-ischemic periods in the brain.
In the eye, as pressure and hypoxia increase, capillary wall failure is more likely and the number, size and distribution of RH increase. Early in the process, RH is manifest as a few, small, central (macular) hemorrhages located in a single layer. But as more capillaries fail, especially in the denser and metabolically demanding areas of the retina, more hemorrhage occurs and blood can coalesce into larger hemorrhages in both the retina and the vitreous. When this occurs, RHs are distributed more widely to the periphery of the eye to the ora serrata, and occur in different layers of the retina. As challenges to the diagnostic reliability of a few central RHs arose after central RH alone appeared in documented non-SBS cases, the criteria to diagnose shaking were changed so that only the more severe forms of intraocular hemorrhage were to be considered diagnostic of nonaccidental injury. Studies by Gilliland52 and Emerson53 document the invalidity of using any pattern as more or less diagnostic of abuse. Emerson found among 118 suspected abuse cases, all forms of eye pathology were present in significant numbers and in different combinations: RH in 44%, folds with schisis cavities in 23% and bilateral in 50% of those cases. Peripapillary scleral hemorrhage was present in 38% of cases, and SDH was present in the distal optic nerve in 46% of cases. Hemosiderin was present in 27% of cases. The variety of findings in abuse did not fit any particular pattern and were distributed almost equally among blunt head injury and blunt injury to the body (52% vs. 40%). Gilliland52 added that “various ocular findings including retinal hemorrhages have been proposed in the literature to unequivocally distinguish non-accidental injuries. Retinal hemorrhages are frequently associated with nonaccidental injuries but other causes must be excluded.” Lantz35 found from autopsy work on 425 eyes of the recently deceased that 17% exhibited RHs associated with a variety of diseases and conditions. It becomes clear that using quantity, location or pattern of RH to diagnose abuse is invalid when extensive RH, often in multiple layers and at times with frank vitreous hemorrhage, is predictably seen in a variety of conditions of extreme ICP, protracted hypoxemia or other conditions.35
Beyond RH, any combination of decreasing perfusion pressure and increasing venous outflow obstruction, both proportional to increased ICP, will result in significant hypoperfusion and hypoxia, damaging more capillaries. The sequence starts in the macula area, dense with capillaries, which leaks first and blood can accumulate under the internal limiting membrane (ILM) the most anterior layer of the retina (Figure 6d). It is here that individual hemorrhages, if sufficient in number, can coalesce to form a pool of blood called a schisis cavity. When these disc-shaped collections of blood pool on the inner surface of the ILM, the blood mass tents the membrane, creating what appear to be retinal folds seen at the edge of the cavity as raised edges (Figure 6a).
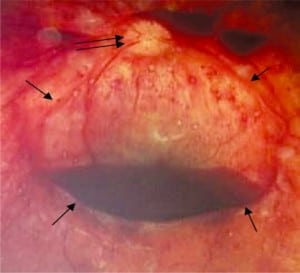
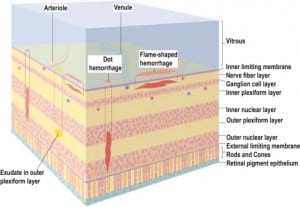
In the most extreme hypoxic states or with DIC, blood overflows these schisis cavities and other areas of dense RH, leading to frank vitreous hemorrhage (Figure 6b) along with more widespread RH to extending to the periphery of the retina. When Blood diffuses into the vitreous and is widely distributed, a cloudy appearance results that can obscure the retinal landmarks (Figure 6c).
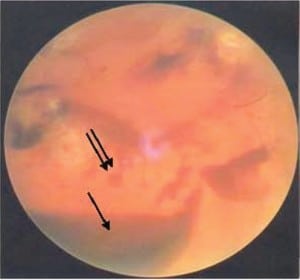
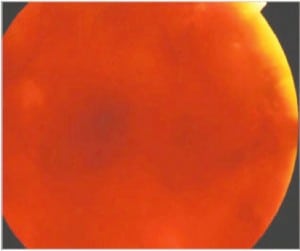
The American Academy of Ophthalmology has endorsed and taught the current corps of ophthalmologists that RH, schisis, retinal folds and vitreous hemorrhage are identified with intentional abuse when in fact these findings are more likely the consequence of metabolic catastrophe within the eye itself and unrelated to shaking forces as discussed above.
Perinatal Subdural Hematoma and its Relation to Shaken Baby Syndrome
The finding of SDH in a neonate, by itself, may not have occurred due to abuse or other types of postpartum trauma. A 2008 study by Rooks54 using MRI to screen “normal” newborns, showed that almost half of newborns have SDH at birth, the result of forces applied to the head during labor. This perinatal SDH (PSDH) was present in 46% of the apparently “normal deliveries.” Extreme molding, cephalohematoma and overriding sutures, common findings after “normal” deliveries, are testament to the forces involved. Protracted labor, pelvic dystocia, the use of oxytocin, prematurity, macrosomy, forceps or vacuum extraction delivery, and vitamin D deficiency (discussed below) are significant risk factors for excess compression of the skull, deformation and dural damage and SDH. With any of these circumstances, PSDH can occur. PSDH, if complicated, in turn can lead to hydrocephalus, subdural hygroma, increased ICP and RH.
When the forces of labor compress the neonatal skull, or protracted hypoxemia occurs for any reason, dural capillaries are damaged and leak as discussed above, forming the PSDH. Newborns must rely on this dural capillary plexus to absorb cerebral spinal fluid (CSF) passing through the porous dural border layer until the arachnoid granulations, which facilitate CSF reabsorption, fully develop around six months of age (Figure 2a). When CSF absorption is blocked by extravasated red blood cells from the PSDH before the arachnoid granulations develop, CSF accumulates between the pia (closely adhered to the brain) and the arachnoid, which remains associated with the dural border cells and the dura. The space that forms is a hygroma. As pressure increases, the hygroma expands and continuous forces are exerted on the unfused skull, resulting in splayed sutures lines and hydrocephalus (Figure 7). Hydrocephalus is the primary clinically measurable complication of PSDH and evolves over weeks to months. It is driven by increased ICP and if the increased ICP is sufficient, RH will develop as discussed above. If the situation does not resolve or stabilize, increased pressure persists and other neuropathic events may occur.
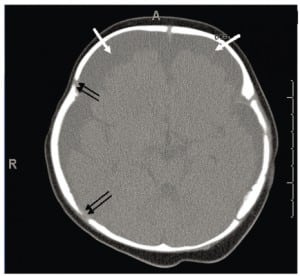
Although more than 99% of neonates with PSDH recover without complications, Rooks described 1/101 (1%) of her subjects who developed these two common complications of PSDH: hygroma and hydrocephalus. The actual complication rate may be as low as .05%, but with about four million births per year in the United States (U.S.) these numbers would still yield 2,000 cases of unresolved PSDH cases with complications. While infrequent, most of these situations lead to a neuropathic event before six months of age that triggers a medical encounter. When the event is frightening, it is considered an apparent life-threatening event (ALTE). The ALTE typically involves apnea, color changes, either erythema, cyanosis, pallor or plethora; changes in muscle tone, usually limpness, but seizures also can occur. Many ALTEs involve choking, gagging and vomiting. At the encounter, any old or new intracranial blood seen on head imaging may generate a working diagnosis of abuse, and initiates a forensic investigation.
Note that in the U.S., healthcare providers are considered “mandatory reporters,” obligated by law to report suspicion of child abuse. Hence, while these findings may be due to rare but described complications of birth trauma, they would invariably result in a report to the local Child Protective Services and/or police agency.
PSDH is generally regarded as asymptomatic at birth, but some neonates have subtle findings that can appear in pediatric outpatient records, if sought. These symptoms and signs may be dismissed as normal postnatal behaviors, such as minor feeding problems, unexpected crying over the first few days after birth, positional problems when breast feeding or a preference to being held upright (to reduce ICP). These findings are virtually never linked to PSDH nor is a specific etiology dependably sought. Newborn ultrasound, done frequently to screen for intracranial bleeding, especially intraventricular hemorrhage, appears incapable of identifying virtually any of the 46% of children born with PSDH and should not be relied on to rule out perinatal intracranial bleeding.54, 55 When such findings are more severe or sufficient to be noted in the record, screening MRI is indicated but virtually never done. Early identification of problematic PSDH with MRI, however, should be regarded as important to investigate subtle behavioral abnormalities to prevent later accusations of abuse if complications arise.
Accelerated head circumference growth, while often missed or ignored, is a relatively reliable clinical indicator of possible complications of PSDH and is readily accessible in most pediatric outpatient records. Early identification of CSDH as a complication of PSDH can lead to appropriate follow-up, more careful handling of such children until the condition resolves and the early detection of potential problems.
Late Complication of Perinatal Subdural Hematoma: Chronic Subdural Hematoma Rebleeds and Apparent Life Threatening Events
If PSDH persists, progresses, or is complicated, neuropathic events occur, including ALTE, usually between six weeks and six months of age.56 This corresponds to the average age bracket in which SBS is diagnosed, 10–13 weeks.57 These ALTE children become the object of intense scrutiny, and child abuse investigations are triggered with any intracranial blood, RH or other bone and soft tissue findings. When SDH and RH, in any form is noted, SBS and intentional abuse is likely to be diagnosed early and other possible etiologies and favorable social factors (no prior violence, long documented records of loving childcare, good character), less seriously considered and frequently disregarded.
When PSDH does not completely resolve, CSDH develops. CSDH is a retracted acute clot infiltrated with fibroblasts and fibrin that achieves structural integrity in the subdural space. (Figures 8a and and8b).8b). This structure can result in “neomembranes” and intradural hemosiderin deposits in or on the inner surface of the dura that can be seen on MRI and at autopsy as free hemosiderin or hemosiderin-laden macrophages. A neomembrane is the result of proliferation and excessive thickening of the normal layer of dural border cells where chronic SDH form and attach.58
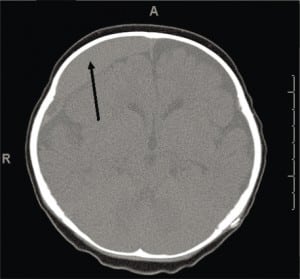
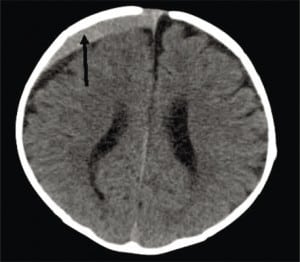
CSDH is a common finding on a first CT of a child presenting with ALTE, who may also have acute or subacute SDH.59 While CSDH may exist without apparent brain damage, it is a bioactive mixture of clotting and declotting factors competing to stabilize and be reabsorbed simultaneously. It is physically fragile and prone to rebleeds with low levels or no apparent force. Rebleeding can occur with a minor trauma (including resuscitative shakes or consoling behaviors), during lumbar puncture or intravenous starts with physical restraint, or crying (Figure 8a), or with normal handling. It is possible that rebleeding of CSDH may be a basis for connecting nonabusive shaking to SDH when mixed collections of old and new blood are seen on imaging and some minor movement event has been reported.
It takes about three weeks for CSDH to appear on CT or MRI, and thereafter the appearance is stable.59 CSDH older than three weeks all appear the same and therefore birth-related bleeding can be present as stable CSDH for some time after birth, generally a number of months to about one year. When imaging demonstrates hematomas of different ages in the same image, there may be no identifiable clinical events to correlate with either a primary or recurrent bleeding. Each episode of rebleeding can elevate already increased ICP, new RH and ALTE may occur, generally before the age of six months.
With premature infants, neurologic events can occur soon after birth, since neuropathic events including apnea spells, dysphagia, and regurgitation, among other neurologic abnormalities, are more frequent with an immature neurologic system. These children with occult birth-related neurologic damage remain quite susceptible to later ALTEs that are frequently not linked to birth-related problems. In spite of very short intervals between the birth problems and the subsequent ALTE, an abuse event may be suspected.
When these infants present after an ALTE, they may have seizures, decreased muscle tone (limpness), vomiting, failure to thrive, hydrocephalus, altered level of consciousness (LOC), color changes from hypoxic episodes, conventional or dysphagic choking, abnormal breathing patterns, and apnea.60 Pediatric records going back to birth may reveal hydrocephalus, irritability, fussiness, and other subtle postnatal problems without explanation, both episodic and recurrent. Examination of perinatal records may be key to identifying subtle signs of PSDH and CSDH, as this may explain the etiology of RH and SDH more plausibly than unwitnessed abusive shaking.
Physical findings of CSDH can include unstable and abnormal vital signs, respiratory instability, including apnea, dysphagia, regurgitation, bulging fontanelle, altered LOC, or seizure activity (focal, generalized or petit mal). RH may be seen immediately by the primary provider, but frequently eye examination is not done until one to two days after presentation. In the interim, ICP may be elevated from a variety of causes and may result in new RH within hours of a precipitous increase in ICP and IOP. RH is expected to be present within 12–36 hours after onset of an event that gradually increases ICP, but if DIC occurs after HIE, sepsis or injury, RH can occur sooner.
With any of these symptoms or physical findings, abuse should be uniformly considered; hence, CT of the head and neck, skeletal surveys and ophthalmology consults are done routinely. Head imaging can show hygroma, hydrocephalus, acute or chronic SDH, or intraventricular or intraparenchymal bleeding. Skeletal survey may reveal metabolic bone disease or prior injury. Eye exam may reveal RH if ICP has been elevated for as little as several hours, if the insult is sufficiently severe. Lab testing can reveal metabolic bone disease, genetic abnormalities, or coagulopathy.
Associated Findings in Suspected Child Abuse Cases
When an infant is found to have RH or SDH, pediatricians routinely perform a skeletal survey to search for corroborative findings of suspected child abuse. However, vitamin D deficiency (VDD) and its bony manifestation, rickets, can lead to deformation of the weakened skull during birth resulting in PSDH, and contribute to bony findings that seemingly confirm the child abuse etiology. VDD and rickets are both widely under-recognized and widespread. In one of the first of a number of studies screening for VDD, 85% of pregnant women in Pittsburgh, screened during labor, were VDD and these deficient levels, as well as any total body calcium deficiency will be passed to the neonate at birth more or less in equal measures.61,62
Skeletal survey frequently reveals various dysplastic bone abnormalities of the extremities and ribs related to VDD rickets. The bony dysmorphic areas seen in rickets may be diagnosed as abusive fractures without consideration of vitamin deficiency. Pathologic fractures may occur as well, as the bones are both soft and weak and generally osteopenic when tested.63
In long bones, the most common rickets-related findings are referred to as classic metaphyseal lesions (CML) and are characterized by child abuse specialists as abusive injuries. These “corner” or “bucket handle” fractures in most alleged abuse cases are painless, have normal range of motion, and no soft tissue injury, making intentional application of force unlikely. The radiographic findings are transient (Figure 9) but may nevertheless be interpreted as abusive, without clinical correlation, vitamin D testing, meaningful differential diagnosis or corroborative history of violence.64
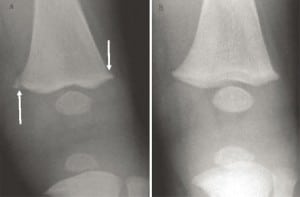
Confusion Between Central Nervous System Infection and Shaken Baby Syndrome
Fever, irritability, meningeal signs, elevated white counts and tense fontanelles suggest central nervous system infection. However, SBS may nevertheless be misdiagnosed for the following reasons. Antibiotics may be given early and empirically, resulting in negative blood cultures. Antivirals are being given empirically as well. Diagnostic lumbar puncture is frequently omitted due to concerns for increased ICP related to SDH. CSF cultures, if done, may be falsely negative after empiric antibiotics, and viral cultures are rarely done on blood and not uniformly on CSF. The self-limited nature of viral meningitis and its low mortality, identified as a cause of ALTE in 1–3% of all ALTE cases, may contribute to missing this diagnosis.56 Chadwick65 estimates that some 30,000 cases of viral meningitis occur in the U.S. per year. While treating “meningitis” prior to laboratory confirmation is certainly a sound clinical strategy, this has substantial legal consequences. Absent or negative cultures due to common early treatment, increases the number of infectious disease cases that go undiagnosed. Absent laboratory evidence of infection, the abuse diagnosis is supported by default. While the presence of RH is an indication for neurologic and ophthalmologic consultation and admission, the presence of RH alone should not be regarded as diagnostic of abuse. Ophthalmologic exam, when increased ICP is present, predictably reveals RH. Absent an obvious medical problem or witnessed accident, the caregiver’s explanation of either a prodromal illness or an accidental injury may be dismissed. The treating physicians relying on the belief that SDH and RH equate to abuse are likely to dismiss other possible etiologies, and meaningful differential diagnosis may cease. The abuse diagnosis is advanced as an increasing certainty in both the medical records and in legal proceedings.
Medical Legal Concerns
Concerns about diagnostic accuracy of SBS are now present and have been recognized by some U.S. courts and the Canadian government.66–67 The Goudge commission, set up in Canada in 2008, investigated SBS and afterwards called for a review and reevaluation of all previous SBS convictions in Canada. The Innocence Project has done a careful and detailed legal and medical analysis further delineating the controversy.68
In response to criticism of the mechanism of SBS, new names have been applied through the years. What began as “shaken whiplash” evolved to SBS. In the 1980s, when biomechanical challenges to the sufficiency of pure human shaking to cause brain injury emerged,6 the concept morphed to encompass “shaken impact.” Now with more evidence disputing the link between shaking and RH, a small group of influential child abuse specialists have advanced the label: “abusive head trauma” (AHT). In April 2009 in a media campaign, SBS was officially rebranded by the American Academy of Pediatrics as AHT, a term crafted to connote an etiology and a legal conclusion and to, in the organization’s words, “provide more clarity in the courtroom.”69–70 While this term’s validity as a medical diagnosis deserves further scrutiny, it does appear to acknowledge that the shaking mechanism is no longer thought sufficient to account for the resultant pathology.
CONCLUSION
According to the evidence presented here, the “prognostic” relationship between shaking and RH is seriously called into question. It appears that SBS does not stand up to an evidenced-based analysis. It appears that RH should not be used as a diagnostic sign of child abuse. It appears that the weight of evidence, both old and new, suggests that increased ICP is a valid, common and predictable cause of RH and that human shaking, by itself, in a healthy child, is insufficient to cause this. Furthermore, children with perinatal SDH, or pre-existing SDH of any cause, are prone to rebleed, resulting in episodes of increased ICP, new RH and more symptoms, which may occur with minimal force applied to the head or with normal handling.
While we must always be mindful of child abuse, which is certainly real and pervasive, we must exercise appropriate restraint to avoid a form of iatrogenic abuse. When children are removed inappropriately from their families, sometimes permanently, based on a specious connection between physical findings and mechanism, there is certainly harm. A detailed history, longitudinal evaluation of the family, true differential diagnosis, and in-depth analysis of all factors discussed here must be done in each case. To do any less is a disservice to our children and their families.
Footnotes
Supervising Section Editor: Paul Walsh, MD, MSc
Submission history: Submitted September 1, 2010; Revision received September 26, 2010; Accepted October 25, 2010
Reprints available through open access at http://escholarship.org/uc/uciem_westjem
Address for Correspondence: Steven C. Gabaeff, MD, FAAEM,FACEP
Email: sgabaeff@adnc.com.
*The chain of physics calculations used is this: Impact velocity, V, resulting from free fall from a given height, h, is: V = SQRT(2*g*h) where g is the force of gravity (32.2 ft/sec2) and h is the height. dV is change in velocity. Average acceleration at impact (a), occurring over a time (dt) with the change in velcoity dV, is a = (dV/dt) OR, average acceleration (a), occurring over a stopping distance, d, can be calculated: a = V2/(2*d). For a 3-foot fall (h = 3 feet), V = 13.9 ft/sec. If we pick a reasonable impact duration of 7.5 msec, then: a = (13.9 / 0.0075) = 1,853 ft/sec2 or 58g and for a velocity of 17 feet/sec then a = (17 / 0.0075) = 2,666 ft/sec2 or 70g. The alternative approach is to pick a reasonable stopping distance of 0.5 inches (0.042 ft) then: a = 13.92 / (2*0.042) = 2300 ft/sec2 or 71g at 3 feet and 106g at 4.5 feet. If we split the difference between the two approaches, that is roughly 65g average acceleration at 3 feet and 90g at 4.5 feet. If we assume a triangular force pulse at impact (standard concept), then the peak values (peak force) are double the average or about 130g peak linear acceleration from 3 feet and 180g at 4.5 feet. The IARV (injury assessment reference value) for the CRABI 6 month ATD (anthropomorphic test device; a type of biofidelic dummy used by the NHTSA) is 50g. To calculate force at impact the mass of the object must be considered. Assuming at head is 4 lbs yields an average force at impact of 265 lbs at 3 ft and about 360 lbs at 4.5 ft. (Calculations validated by John D. Lloyd, PhD, CPE).
Conflicts of Interest: By the WestJEM article submission agreement, all authors are required to disclose all affiliations, funding sources, and financial or management relationships that could be perceived as potential sources of bias. The authors discloses he is a designated expert by the Los Angeles County Committee of Superior Court Judges “appointed to maintain a Panel of Expert Wittnesses”, “providing expertise for both prosecutors and defense attorneys” in the areas of emergency medicine, child abuse and sexual assault. The author has provided expert opinions on medical-legal issues in criminal, civil and dependency matters for over 22 years in more than 1,300 consultations.
REFERENCES
1. Donohoe M. Evidence-Based Medicine and Shaken Baby Syndrome Part I: Literature Review, 1966–1998. Am J Forensic Med Pathol. 2003;24:239–42. [PubMed]
2. Caffey J. The whiplash shaken infant syndrome: manual shaking by the extremities with whiplash-induced intracranial and intraocular bleedings, linked with residual permanent brain damage and mental retardation. Pediatrics. 1974;54:396–403. [PubMed]
3. Guthkelch AN. Infantile subdural hematoma and its relationship to whiplash injuries. Brit Med J.1971;2:430–1. [PMC free article] [PubMed]
4. Ommaya AK, Faas F, Yarnell P. Whiplash injury and brain damage: an experimental study. JAMA.1968;204(4):285–9. 22; [PubMed]
5. Klinich K, Hulbert G, Schneider L. Estimating infant head injury criteria and impact response using crash reconstruction and finite element modeling. Stapp Car Crash Journal. 2002;46
6. Duhaime AC, Gennarelli TA, Thibault LE, et al. The shaken baby syndrome. A clinical, pathological, and biomechanical study. J Neurosurg. 1987;66:409–15. [PubMed]
7. Ommaya AK, Gennarelli TA. Cerebral concussion and traumatic unconsciousness. Correlation of experimental and clinical observations of blunt head injuries. Brain. 1974 Dec;97(4):633–54.[PubMed]
8. Ommaya AK, Hirsch AE, Flamm ES, et al. Cerebral concussion in the monkey: an experimental model. Science. 1966;153(3732):211–2. [PubMed]
9. King W, MacKay M, Sirnick A. With the Canadian Shaken Baby Study Group Shaken baby syndrome in Canada: clinical characteristics and outcomes of hospital cases. CMAJ. 2003;168(2):155.[PMC free article] [PubMed]
10. Bandak FA. Shaken baby syndrome: A biomechanics analysis of injury mechanisms. Forensic Science International. 2005;151:71–9. [PubMed]
11. Commonwealth v Ann Power Report to the Middlesex County District Attorney’s Office Cambridge Massachusetts by Carole Jenny dated December 29, 2005. Letters. 2005;319
12. Prange MT, Coats B, Duhaime A-C, Margulies SS. Anthropomorphic simulations of falls, shakes, and inflicted impacts in infants. J Neurosurg. 2003;99:143–50. [PubMed]
13. Barnes PD. Child Abuse – Nonaccidental Injury (NAI) Issues and Controversies for Neuroradiology in the Era of Evidence-Based Medicine-Article submitted for review. 2009.
14. Molina DK., Neck Injuries and Shaken Baby Syndrome Letter to the editor. American J of Forensic Med and Pathology. 2009 Mar;30(1):89.
15. Duncan JM. Laboratory Note: On the Tensile Strength of the Fresh Adult Foetus. BMJ.1874;2:763–4.
16. Leestma JE. SBS and confessions. J Am Phys Surg. 2006
17. Case ME, Graham MA, Handy TC, et al. National association of medical examiners ad hoc committee on shaken baby syndrome. Position paper on fatal abusive head injuries in infants and young children. Am J Forensic Med Pathol. 2001;22(2):112–22. [PubMed]
18. Funk JR, Duma SM, Manoogian SJ, et al. Biomechanical risk estimates for mild traumatic brain injury. Annu Proc Assoc Adv Automot Med. 2007;51:343–61. [PMC free article] [PubMed]
19. Raghupathi R, Margulies SS. Traumatic axonal injury after closed head injury in the neonatal pig.J Neurotrauma. 2002;19:843–5. [PubMed]
20. Binenbaum G, Forbes BJ, Raghupathi R, et al. An animal model to study retinal hemorrhages in nonimpact brain injury. J AAPOS. 2007;11:85–5. (Abstract).
21. Greenes DS, Schutzman SA. Infants with isolated skull fracture: what are their clinical characteristics, and do they require hospitalization. Ann Emerg Med. 1997 Sep;30(3):253–9.[PubMed]
22. Greenes DS, Schutzman SA. Occult intracranial injury in infants. Ann Emerg Med. 1998 Dec;32(6):680–6. [PubMed]
23. Plunkett J. Fatal Pediatric Head Injuries Caused by Short-Distance Falls. Am J Forensic Med and Pathology. 2001;22(1):1–12.
24. Lettau M, et al. 3T High-spatial-resolution contrast-enhanced MR angiography of the intracranial venous system with parallel imaging. Am J Neuroradiol. 2009;30:185–7. [PubMed]
25. Maxeiner H. Demonstration and interpretation of bridging vein ruptures in cases of infantile subdural bleedings. J Forensic Sci. 2001;46:85–93. [PubMed]
26. Squier W. Shaken baby syndrome: the quest for evidence. Dev Med Child Neurol. 2008 Jan;50(1):10–4. [PubMed]
27. Morimoto T, et al. Monitoring venous blood flow velocity during interhemispheric approach to deep seated lesions. Acta Neurochirurggica. 1995;137:44–7.
28. Mack J, Squier W, Eastman JT. Anatomy and development of the meninges: implications for subdural collections and CSF circulation. Pediatr Radiol. 2009 Mar;39(3):197–8. [PubMed]
29. Vinchon M, Delestret I, DeFoort-Dhellemmes S, et al. Subdural hematoma in infants: can it occur spontaneously? Data from a prospective series and critical review of the literature. Childs Nerv Syst.2010 epub ahead of print.
30. Cohen MC, Scheimberg I. Evidence of occurrence of intradural and subdural hemorrhage in the perinatal and neonatal period in the context of hypoxic ischemic encephalopathy: An observational study from two referral institutions in the United Kingdom. Ped and Develop Pathology.2009;12:169–76.
31. Syrogiannopoulos G, Nelson JD, McCracken GH., Jr Subdural collections of fluid in acute bacterial meningitis: a review of 136 cases. Pediatr Infect Dis. 1986;5:343. [PubMed]
32. Chadwick DL, Bertocci G, Castillo E, et al. Annual risk of death resulting from short falls among young children: less than 1 in 1 million. Pediatrics. 2008 Jun;121(6):1213–24. [PubMed]
33. Taylor AS. Medical Jurisprudence, Blanchard and Lea. 4th Edition. Philadelphia: 1856. pp. 366–70.
34. Goldsmith W, Plunkett J. Biomechanical analysis of the causes of traumatic brain injury in infants and children. Am J Forensic Med Pathol. 2004;25:89. [PubMed]
35. Lantz PE, Sinal SH, Staton CA, et al. Evidence based case report: perimacular retinal folds from childhood head trauma. BMJ. 2004;328:754–6. [PMC free article] [PubMed]
36. Obi E, Watts P. Are there any pathognomonic signs in shaken baby syndrome? J AAPOS.2007;11:99–100.
37. Christian CW, Taylor AA, Hertle RW, et al. Retinal hemorrhages caused by accidental household trauma. J Pediatr. 1999 Jul;135(1):125–7. [PubMed]
38. Terson PDA. Hemorrhage in the vitreous body during cerebral hemorrhage. La Clinique Ophthalmologique. 1900;22:309–12.
39. Medele RJ, Stummer W, Mueller AJ, et al. Terson’s syndrome in subarachnoid hemorrhage and severe brain injury accompanied by acutely raised intracranial pressure. J Neurosurg. 1998 May;88(5):851–4. [PubMed]
40. Reddy AR, Clarke M, Long VW. Unilateral retinal hemorrhages with subarachnoid hemorrhage in a 5-week-old infant: is this nonaccidental injury? Eur J Ophthalmol. 2010 Jan 5;20(4):799–801.[PubMed]
41. Walsh FB, Hedges TR. Optic nerve sheath hemorrhage. Am J Ophthalmol. 1951;34:509–27.[PubMed]
42. Muller PJ, Deck JHN. Intraocular and optic nerve sheath hemorrhage in cases of sudden intracranial hypertension. J Neurosurg. 1974;41:160–6. [PubMed]
43. Aoki N, Masuzawa H. Infantile acute subdural hematoma: clinical analysis of 26 cases. J Neurosurg. 1984;61:273–80. [PubMed]
44. Lashutka MK, Chandra A, Murray HN, et al. The relationship of intraocular pressure to intracranial pressure. Ann Emerg Med. 2004;43:585–91. [PubMed]
45. Lantz PE, Stanton CA. Postmortem detection and evaluation of retinal hemorrhages. Am Acad Forens Sci; Abstract, presented at the AAFS Annual meeting; Seattle, WA. February 2006.2006.
46. Tayal VS, Neulander M, Norton HJ, et al. Emergency department sonographic measurement of optic nerve sheath diameter to detect findings of increased intracranial pressure in adult head injury patients. Ann Emerg Med. 2007;49:508–514. [PubMed]
47. Talbert D. Paroxysmal cough injury, vascular rupture and ‘shaken baby syndrome’ Medical Hypotheses. 2005;64:8–13. [PubMed]
48. Duszak RS. Retinopathy, valsalava. 2009. Available at:http://emedicine.medscape.com/article/1228106-overview.
49. Hornbein TF, Schoene RB. High altitude: an exploration of human adaptation. Marcel Dekker Inc., NY; NY: 2001. p. 744.
50. Reece RM, Nicholson CE. Inflicted childhood neurotrauma. In: Reece RM, Nicholson CE, editors. Proceedings of a conference sponsored by HHS, NIH, NICHD, ORD, NCMRR; 02 Oct 10; American Academy of Pediatrics; 2003.
51. Koto T, et al. Hypoxia Disrupts the Barrier Function of Neural Blood Vessels through Changes in the Expression of Claudin-5 in Endothelial Cells. Am J of Pathology. 2007;170:1389–97.
52. Gilliland M. Why do histology on retinal haemorrhages in suspected non-accidental injury?Histopathology. 2003;43:592–602. [PubMed]
53. Emerson M, Jakobs E, Green R. Ocular Autopsy and Histopathologic Features of Child Abuse.Ophthalmology. 2007;114:1384–94. [PubMed]
54. Rooks VJ, Eaton JP, Ruess L, et al. Prevalence and evolution of intracranial hemorrhage in asymptomatic term infants. Am J Neuroradiol. 2008 Jun;29(6):1082–9. [PubMed]
55. Chambers S, Hendry G, Wild S. Real time ultrasound scanning of the head in neonate and infants, including correlation between ultrasound and computed tomography. Pediatric Radiology.1985;15:4–7. [PubMed]
56. Mittal MK, et al. Serious Bacterial Infections in Infants who have Experienced and Apparent Life-threatening Event. Annals of Emergency Medicine. 2009 Oct;54(4):523–7. [PubMed]
57. Barr RG, Roger BT, Cross J. Age-related incidence curve of hospitalized shaken baby syndrome cases: convergent evidence for crying as a trigger to shaking. Child Abuse & Neglect. 2006;30(1):7–16. [PubMed]
58. Friede RL, Schachenmayr W. The origin of subdural neomembranes. II. Fine structural of neomembranes. Am J Pathol. 1978 Jul;92(1):69–84. [PMC free article] [PubMed]
59. Vezina G. Assessment of the nature and age of subdural collections in nonaccidental head injury with CT and MRI. Pediatr Radiol. Published online 21 Mar 2009.
60. Till K. Subdural Haematoma and Effusion in Infancy. Brit Med J. 1968;3:400–2. [PMC free article][PubMed]
61. Bodnar LM, et al. High prevalence of vitamin D insufficiency in black and white pregnant women residing in the northern United States and their neonates. J. Nutr. 2007;137:447–52. [PubMed]
62. Ziegler E, Hollis B, Nelson S, et al. Vitamin D deficiency in breastfed infants in Iowa. Pediatrics.2006;118(2):603–10. [PubMed]
63. Park E, Howland J. The dangers to life of severe involvement of the thorax in rickets. Bulletin of the Johns Hopkins Hospital. 1921;32:362.
64. Keller KA, Barnes PD. Rickets vs. abuse: a national and international epidemic. Pediatr Radiol.2008 Nov;38(11):1210–6. [PubMed]
65. Chadwick DR. Department of Infection & Travel Medicine, The James Cook University Hospital; Middlesbrough: Viral meningitis. Published Online February 10, 2006.
66. Commonwealth of Kentucky (plaintiff) vs. Christopher A. Davis (defendant), Case No. 04-Cr-205. Order And Opinion, Re: Daubert Hearing. Commonwealth Of Kentucky, Greenup Circuit Court Filed 4-17-06.
67. Cordner S, Ehsani J, Bugeja L, Ibrahim J. Ontario, Canada: Nov 28, 2007. Pediatric forensic pathology: limits and controversies. Commissioned by the Inquiry into Pediatric Forensic Pathology, Available at:http://www.goudgeinquiry.ca/policy_research/pdf/Limits_and_Controversies-CORDNER.pdf.
68. Tuerkheimer D. The Next Innocence Project: Shaken Baby Syndrome And The Criminal Courts.Washington University Law Review. 2009;87(1)
69. Christian CW, Block R., the Committee on Child Abuse Abusive Head Trauma in Infants and Children. Pediatrics. 2009;123:1409–11. [PubMed]
70. Associated Press “Doctors recommend new term for ‘shaken baby syndrome’.” Boston Globe. 4-27-09 Available at:http://www.boston.com/news/nation/articles/2009/04/27/doctors_recommend_new_term_for_shaken_baby_syndrome/